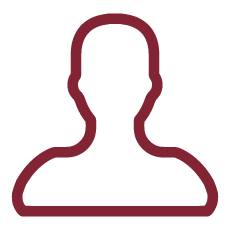
At the few-atom-thick limit, transition-metal dichalcogenides (TMDs) exhibit strongly interconnected structural and optoelectronic properties. The possibility to tailor the latter by controlling the former is guaranteed to have a great impact on applied and fundamental research. As we recently discovered, hydrogen (ions) irradiation deeply affects the surface morphology of bulk TMD crystals. H+ ions penetrate the top layer, resulting in the TMD-catalyzed production and progressive accumulation of molecular hydrogen in the first interlayer region. This leads to the blistering of one-monolayer thick domes, which stud the crystal surface and locally turn the dark bulk material into an efficient light emitter. The domes are stable and robust, and host strong, complex strain fields. Lithographic techniques allow to engineer the formation process so that the domes can be produced with well-ordered positions and sizes tunable from the nanometer to the micrometer scale.
The final goal of the "Advanced Nanophotonics based on Hydrogenated Transition-Metal Dichalcogenides (ANaHTraMeDi)" project is the development of a unique platform for the realization of scalable, advanced light emitters, based on single, strain-engineered TMD nanodomes. Such nanodomes will be deterministically integrated in circular Bragg grating (bullseye) cavities, which have shown excellent performances in maximizing the extraction efficiency of the photons emitted by semiconductor nanostructures. Three main device types will be targeted throughout the course of the project: (i) Nanolasers; (ii) Bright single- (and entangled-)photon emitters; (iii) Cavities for the enhancement of the efficiency of nonlinear frequency conversion via Second-Harmonic Generation (SHG). Towards the end of the project we will also aim at achieving full dynamic control of the properties of these devices via strain engineering, by (iv) integrating them with piezoelectric (PZT) actuators.
At present, two of the main funding initiatives undertaken by the European Union place a strong focus on condensed matter physics and materials science. The first program, the Future and Emerging Technologies (FET) Flagship on Graphene (see https://graphene-flagship.eu/project/Pages/About-Graphene-Flagship.aspx) is currently in the second half of its planned ten-year run (2013-2023), and devotes a ~€1 billion budget to the goal of "bringing together academic and industrial researchers to take graphene [and related 2D materials] from the realm of academic laboratories into European society [...], thus generating economic growth, new jobs and new opportunities". The second initiative, the FET Flagship on Quantum Technologies (http://ec.europa.eu/research/participants/portal/desktop/en/opportunitie...) was launched last year with the aim of moving "advanced quantum technologies from the laboratory to industry with concrete prototype applications and marketable products while advancing at the same time the fundamental science basis".
By aiming at the realization of advanced light sources based on the integration of site-controlled TMD nanodomes with bullseye cavities, possibly relying on strain engineering to dynamically control the device properties, the present project sits squarely at the intersection between these two Flagship initiatives. On the one hand, as reported in §2.8 and 2.9 of the "Science and technology roadmap for graphene, related two-dimensional crystals, and hybrid systems" [Fer15], an important goal of the Graphene Flagship is indeed represented by the identification, characterization and practical exploitation of 2D materials alternative to graphene. As noted in the previous sections, transition metal dichalcogenides hold a preeminent place within this context, due to their huge potential for light-emitting applications. The development of the ability to harness the effects of strain -and of quantum confinement, for domes smaller than 10-20 nm- on the electronic and optical properties of 2D TMD micro- and nano-domes will represent a crucial breakthrough in the field, paving the way to the exploitation of these effects for the fine tuning of the light-emitting properties of the fabricated nanodevices.
This latter goal is also what establishes a strong link between the present project and the newly launched Flagship on Quantum Technologies. As noted in the Final Report of the High-Level Steering Committee of the Quantum Technologies Flagship (https://tinyurl.com/QT-HLSC-report), the development of bright, site-controlled single-photon sources with tuneable properties -possibly compatible with current, Si-based, optoelectronic devices- would be beneficial to many of the overarching goals of the Flagship, such as the development of Quantum Simulators with a certified quantum advantage (wherein ordered arrays of identical single-photon sources will likely be required) and the establishment of commercially viable protocols for Quantum Communications. Within this framework, the ultimate goal of the present project, i.e., the development of possibly room-temperature, strain-tuneable single-photon sources based on size- and position-controlled nanodomes, would be hailed as a major milestone. This is particularly true at the light of the inherent integrability of these nanodomes with photonic devices (see Fig. 1). As noted above, such integration will allow us to further control and optimize the properties of our light emitters -particularly in terms of their radiative recombination rate (Purcell Effect ) and light extraction efficiency [Liu19]- eventually enabling the realization of advanced light-emitting devices, e.g., nanolasers [Sch05] and photonic devices based on non-linear effects. The latter are particularly appealing for integrated optics, relying on light control modalities that include optical switching, phase control, and frequency conversion, e.g., SHG [Moh17].