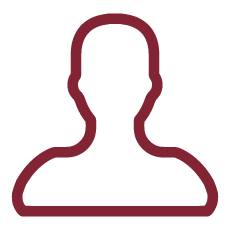
Gels are highly porous disordered solids which play a crucial role in several biological systems. Gels can arise in suspensions of colloidal particles or solutions of macromolecules which organize themselves into a disordered structure as a result of very specific attractive interactions. Within this project we plan to explore new pathways to physical gelation in bio-inspired systems in which the bond strength is comparable to the thermal energy (reversible bonds). Specifically we will focus on DNA-based gelling systems, such as DNA stars, G-quartets and DNA-based vitrimers. The fundamental issues which will be addressed in our investigations concern (i) the structural and dynamical features related to the formation and breaking of reversible bonds in these gels; (ii) the dependence of rheological and mechanical properties of the gel on network topology and on strength and lifetime of reversible bonds and (iii) how to design the DNA particles to encode in the resulting material the desired mechanical and thermal properties, along the lines of DNA-nanotechnology. We will conduct a combined experimental and computational investigation,
merging the potential provided by computational approaches by carrying out simulations of full atom or accurate coarse-grained model with neutrons and light scattering experiments.
By fully characterizing the gelation mechanisms in these systems and the rich and complex dynamics of networks stabilized by reversible bonds we hope (i) to provide a deeper understanding of the factors influencing the gel stability and (ii) to elucidate the connections between the designed gel porous structure and the peculiar rheological and mechanical properties of these soft materials. Finally, we hope to provide new insights in the design of novel DNA based nanoconstructs relevant for material science and biomedical applications.
The research in material science has recently witnessed a strong effort to design hierarchical soft materials with a bottom-up approach borrowing tips and tricks from biological structures. Among them, hydrogels play a key role due to their unique softness, resilience, and biocompatibility. It is precisely their bottom-up structuring that makes soft networks unique candidate for the design of adaptive materials [7,8], capable of responsiveness to external stimuli, self-healing abilities, resiliency (ability to mantain their network topology despite large strains) and controllable rheomechanical properties. By addressing these pliable systems, we expect several aspects of this project to impact on science advancement and to provide new strategies to tackle technological challenges. By performing a wide range survey of diverse hydrogels differing in building blocks route to gelation (equilibrium vs. nonequilibrium), structure (supramolecular, self-assembled, composite, heterogeneous), and physical properties (microscopic dynamics, rheology, response to perturbation), we expect to single out the key distinctive traits shared by these materials and to pinpoint the peculiar features of each class of hydrogels. In fact, aim of the present project is providing the soft matter community with a new insightful perspective of these systems.
Given the disruptive potential of the design and engineering of functional hydrogels for multifarious material science and bioclinical applications, we are also confident that we will be able to indicate how to exploit these smart materials. We shall mainly focus on three practical issues, namely engineering hydrogels in which viscoelasticity is given by design and/or sample preparation, producing networks with catalyst-controlled rheological and self-healing properties, designing surface-bound gels responsive to specific molecular species.
In other words, we plan to expand the design of available hydrogels, bringing it to a new level of complexity without however losing the advantage intrinsic to self-assembly. Two new design concepts will be introduced: (i) interpenetrated - but still water-rich - networks, which we expect to show a remarkable resilience and possible new mechanic features due to the topological constraints intrinsic to their microscopical interprenetration. (ii) hydrogels with composition gradients encrypted in the structure by tuning the gelation kinetics via a controlled enzymatic dissociation of a chemical agent that induces a pH or ionic strength change. Besides playing an important role in living systems, gels with a spatially-modulated structure are at the forefront of many bioclinical applications. One of the key practical issues to obtain them is accurately tuning the gelation kinetics with respect to the perfusion time of a gelling agent.
We shall explore the possibility to create a self-healing plastic entirely made of DNA oligomers. By controlling the temperature in a limited interval around room temperature, the network of bonds can restructure itself or can be essentially frozen. This new bio-compatible material will not only self-assemble in a stress-free configuration, but will also be able to self-heal by progressively swapping broken bonds with new ones.
Furthermore, we wish to pave the way for creating new - smart surface hydrogels - that could be used as a transducers for the recognition of specific molecular species in the solution contacting the surface. They will be built developing on recently proposed strategies for the exponential growth of multi-strand nucleic acid clusters [19,20]. This strategy can be exploited, on hydrogels built on surfaces, whose production involves minimal amount of chemicals and reduced reaction time. Such smart surface hydrogel could have significant applications in all surface-based biodetectors. We will aim at designing a proof-of-concept for a simple method for the production of such gels, which can be tested experimentally. Indeed, within the limited timescale of the present project in condensed matter physics, our minimal goal is giving at least a proof-of-concept of these new ideas through coarse-grained and all-atom computer simulations. A distinctive feature of our approach, however, is that exploiting will be strongly rooted on the concepts and methods developed by exploring.
REFERENCES
see "Descrizione obiettivi progetto" and "Inquadramento della ricerca"