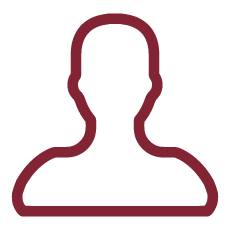
Natural Killer (NK) cells represent a pivotal player of innate immune responses, and may also positively or negatively affect the development, breadth and persistence of adaptive responses. NK cells may contribute to immune homeostasis and to the pathogenesis of autoimmune diseases by producing cytokines and chemokines, and through direct cell-cell interactions with dendritic cells (DC) and T cells. The impact of environmental factors on NK cell compartment is increasingly appreciated. Human Cytomegalovirus (HCMV) infection induces the expansion of a long-lived, phenotypically heterogeneous, "memory" NK cell population, characterized by enhanced CD16 (low affinity receptor for IgG)-dependent functional capabilities, in a fraction of seropositive subjects. The mechanisms that regulate memory NK cell pool size, long-term persistence, and activation have not been fully characterized yet. Chronic interaction with IgG-opsonized cells may represent an efficient stimulus for memory NK cell expansion and/or maintenance in vivo. We have recently described the perturbation of the equilibrium of the memory NK cell subsets in a cohort of HCMV+ patients affected by immune thrombocytopenia (ITP), an autoimmune disease where anti-platelet autoantibodies play a major role. We have also found that ITP, but not control, platelets selectively promote memory NK cell in vitro expansion. Memory NK cells are placed in a privileged position for exerting a regulatory role in antibody-dependent autoimmune diseases. In the present project, we will analyze several aspects of the functional profile of memory NK cell subsets in ITP patients. We will focus on cell surface and soluble molecular signals that may dictate the crosstalk of memory NK cells with DC and T cells, such as: a) the expression of immune checkpoint repertoire, b) the molecular signature relevant for survival, self-renewal advantage, and susceptibility to apoptosis, c) CD16-dependent effector functions.
No information exist in the literature on the possible dysregulation of memory NK cells in autoimmune condition; moreover, whether their CD16-dependent immunoregulatory functions may contribute to amplify/sustain, or rather be relevant for limiting/dampening auto-aggressive immune responses is unknown. The results of our study will define the possible functional alterations of the memory NK cell compartment in ITP patients, and will assess the impact of persistent stimulation with autoantibody-coated target cells on memory NK cell functional response. These novel information will:
a) provide foundation knowledge for further studies aimed at evaluating more in depth the functional outcomes of the interaction between memory NK cells and autoantibody-coated cells, to assess their contribution to autoimmune tissue damage;
b) be a prerequisite for analyzing the impact of memory NK cell effector functions on autoreactive T and B cells;
c) provide a rational basis for further studies aimed at the therapeutical manipulation of memory NK cells in autoimmune diseases.
References
1. McCarville JL, et al. Annu Rev Immunol. 2020;38:147-170.
2. Zhao Q, et al. Immunology. 2018;154(1):28-37.
3. Ruff WE, et al. Nat Rev Microbiol. 2020; 18(9):521-538.
4. Goodrum F. Annu Rev Virol. 2016;3(1):333-357.
5. Dreyfus DH. J Allergy Clin Immunol. 2013;132(6):1278-86.
6. Pawelec G. Exp Gerontol. 2014;54:1-5.
7. Davis MM, Brodin P. Annu Rev Immunol. 2018;36:843-864.
8. Cruz-Munoz ME. Front Microbiol. 2018;8:2521.
9. Vivier E, et al. Science. 2011;331(6013):44-9.
10. Barrow AD, et al. Front Immunol. 2019;10:909.
11. Mace EM, et al. Immunol Rev. 2019;287(1):202-225.
12. Vivier E et al. Cell. 2018;174(5):1054-1066.
13. Trinchieri G, et al. Nat Immun. 1993;12:218-34.
14. Long EO, et al. Annu Rev Immunol. 2013;31:227-58.
15. Warren HS, et alF. J. Immunol. 1999;162:735-42.
16. Lee HR, et al. Sci Rep. 2017;7:11075.
17. Gyurova IE, et al. Viral Immunol. 2020;33(4):334-341.
18. Pallmer K, Oxenius A. Front Immunol. 2016;7:25.
19. Schuster IS et al. Front Immunol. 2016;7:2351.
20. Toubi E et al. Autoimmun Rev. 2019;18(3):306-311.
21. Smith SL, et al. Blood Adv. 2020;4(7):1388-1406.
22. Sun JC, et al. Cold Spring Harb Perspect Biol. 2018;10(10):a029538.
23. Capuano C, et al. Front Immunol. 2018;9:1031.
24. Rölle A, et al. Trends Immunol. 2016;37:233-43.
25. Cerwenka A, et al. Nat Rev Immunol. 2016;16:112-23.
26. Tesi B, et al. Trends Immunol. 2016;37:451-61.
27. Guma M, et al. Blood. 2004;104:3664¿71.
28. Lopez-Verges S, et al. Proc Natl Acad Sci USA. 2011;108:14725¿32.
29. Della Chiesa M, et al. Blood. 2012;119:399¿410.
30. Foley B, et al. Blood. 2012;119:2665¿74.
31. Beziat V, et al. Blood. 2013;121:2678¿88.
32. Schlums H, et al. Immunity. 2015;42:443¿ 56.
33. Lee J, et al. Immunity. 2015;42:431-42.
34. Pfefferle A, et al. Front Immunol. 2020 May;11:812.
35. Zhang C, et al. J Autoimmun. 2017;83:22-30.
36. Zitti B, et al. Cytokine Growth Factor Rev. 2018;42:37-46.
37. Beziat V, et al. Blood. 2013;121 (14):2678¿2688.
38. Al-Samkari H, et al. Semin Thromb Hemost. 2020;46(3):275-288.
39. Lambert MP, et al. Blood. 2017;129(21):2829-2835.
40. Cooper N, et al. Br J Haematol. 2006;133(4):364-374.
41. Zufferey A, et al. J Clin Med. 2017;6(2):E16.
42. Olsson B, et al. Nat Med. 2003;9(9):1123-4.
43. Cooper N, et al. N Engl J Med. 2019;381(10):945-955.
44. Agrawal S, et al. Clin Immunol. 2020;216:108449.
45. Nourse JP, et al. Blood Coagul Fibrinolysis. 2012;23(1):45-50.
46. Ebbo M, et al. Clin Immunol. 2017;177:18-28.
47. Talaat RM, et al. Clin Exp Immunol. 2014;176(2):291-300.
48. Monzón Manzano E, et al. Br J Haematol. 2020;189(5):943-953.
49. Zhao Z, et al. PLoS One. 2015;10(5):e0126601.
50. Zhang J, et al. Hematology. 2018;23(8):510-516.
51. Neunert C, et al., Blood. 2011;117(16):4190-4207.
52. Provan D, et al. Blood Adv. 2019;3(22):3780-3817.
53. Neunert C, et al. Blood Adv. 2019;3(23):3829-3866.
54. Birocchi S, et al. Platelets. 2020;1-11.
55. Sivori S, et al. Front Immunol. 2020;3;11:2156.
56. Pauken KE, et al. Semin Immunol. 2021;101480. Online ahead of print. doi: 10.1016/j.smim.2021.101480.