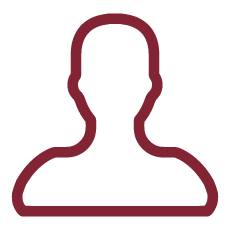
The growing global energy consumption and civil transportation hinges ever more heavily on the combustion of fossil fuels. In addition, health-related issues of air pollution, impose strict constraints on combustion emissions and set stringent requirements on the efficiency of devices, whether for aeronautical or power generation applications. This calls for an ever growing presence of fundamental research in combustion science. It is therefore essential to recognize the role of turbulent premixed flames as a ubiquitous and primary constituent of combustion chamber phenomenology. Indeed, the underlying chamber turbulent flowfield, typically encompassing a spectrum of spatial and temporal scales, interacts with the propagating structure of premixed flames, giving rise to a complex fenomenology which is an extremely active and wide area of experimental, numerical and theoretical research.
Our research group at the Dept. of Mechanical and Aerospace Engineering at La Sapienza has a long standing experience in combustion research which ranges from the diagnostics of complex reactive fields, to the generation of reduced chemical kinetics mechanisms for higher hydrocarbons, to the numerical simulation of turbulent reactive fields.
In this respect, the group has been actively pursuing highly computationally intensive simulations of premixed turbulent flames with the objective of analyzing the role of domain size or, equivalently, flame scale, on their morphological and propagative features. This extremely novel area of research has proved very promising in unveiling new phenomenology which typically went undetected given the reduced domain size of state of the art direct numerical simulations.
In light of the promising features of this research we put forth this proposal with the objective of extending the scale of our current simulations and, concurrently, to ensure continuity to these activities in terms of development of more effective numerical infrastructures.
Turbulent premixed combustion is an intrinsically multiscale physical phenomenon, encompassing fluid dynamic scales as small as the Kolmogorov dissipative scales and as large as the largest scales of the combustion device under investigation. An ever present scale is also the local flame thickness which, depending on the Kolmogorov scale and the Reynolds number, may represent the smallest physical scale. Temporal scales play an equivalent role, with eddy turnover times and chemical kinetic timescales representing the multiscale temporal nature of the phenomenon.
The current petascale computational applications allow for the numerical representation of such turbulent premixed combustion phenomena at the continuum level. Indeed, up to 4 decades of scales can be encompassed by any petascale-class numerical simulation. Direct numerical simulations (DNS) usually encompass micron to millimeter scales without the need for subgrid modelling, Large Eddy Simulations (LES) encompass 10-100 micron to 10-100 millimiter scales while Reynolds Averaged Navier-Stokes (RANS) approaches encompass millimeter to meter scales.
While LES techniques resolve up to 80% of the turbulent kinetic energy and need sub-grid modeling of the unresolved small scales, RANS approaches, which can reproduce device-scale phenomena useful in engineering applications, do not directly resolve any fluid dynamic scale and thus need a full-range of scale modelling. The effectiveness and reliability of sub-grid models, clearly rests on the information that can be gleaned from DNS data. The underlying assumption, however, is that premixed flames are not subject to any "large scale" phenomenon as they will go undetected by DNS due to the domain size limitation on the largest scale.
As we have seen, however, premixed flames are indeed subject to large scale effects, in the form of long-wavelength or Darrieus-Landau instabilities which induces additional forms of corrugation on the flame, thereby deeply affecting its morphology. The larger
the flame scale (i.e. domain size) the wider the spatial range of corrugation induced, which can acquire a self-similar or fractal nature [10,17].
In this context, the analysis of large scale effects in a DNS context is therefore of great importance as it will lead to the formulation of new sub-grid models for LES and RANS. This is also true in the presence of hydrogen-rich mixtures which are likely to give rise to additional instabilities of thermal-diffusive nature. For these reasons, the present research is likely to be transformative in the context of premixed modeling as it promises to include physical phenomena which have been overlooked to the present day and are absent in the current state of the art.
Indeed, among the main future issues in turbulent premixed combustion, Sabelnikov and Lipatnikov, in a very recent review [18], clearly underline that "In DNS of premixed turbulent flames, the influence of the computational domain width is worth studying to gain insight into eventual Darrieus-Landau instability of the entire turbulent flame brush."
The proposed research program will also take advantage of especially designed premixed flame experiments, in a Bunsen burner configuration, carried out at ENEA Casaccia Combustion Research laboratory under the supervision of Dr. Guido Troiani. Results from
such experiments have already been included in the group's recent publications [15,16] and have played an important role in validating all DNS results.
[1] Damkohler, G., Z. Elektrochem. 46, 601-652 (1940)
[2] Darrieus, G., 1945 at Congres de Mecanique Appliquee (Paris).
[3] Landau, L. D., Acta Physicochim. USSR 19, 77-85 (1944).
[4] J. H. Chen, Proc. Combust. Inst. 33, 99-123 (2011).
[5] H. Wang et al., Proceedings of the Combustion Institute, 36(2):2045-2053, (2017).
[6[ P. Trisjono, Proc. Combust. Inst., 36(2):2033¿2043, (2017).
[7] C. Towery, et al., Physical Review E, 93(5):053115, 2016.
[8] B. Savard et al., Proc. Combust. Inst., 35(2):1377-1384, (2015).
[9] Creta, F., Matalon, M., Proc. Combust. Inst. 33 (2011) 1087-1094.
[10] Creta, F. et al., Combustion Theory and Modelling} 15(2) (2011) 267-298.
[11] Creta, F., Matalon, M., Journal of Fluid Mechanics 680 (2011), pp. 225-264.
[12] N. Fogla et al., Proc. Combust. Inst. 34 (2013), pp. 1509-1517.
[13] N. Fogla et al., Cobustion and Flame 162 (2015), pp. 2758-2777.
[14] N. Fogl et al., Cobustion and Flame 175 (2017), pp. 155-169.
[15] Troiani, G., et al.., Proc. Combust. Inst. 35 (2015), pp. 1451-1459.
[16] Creta, F., Lamioni, R., Lapenna, P.E., Troiani, G., Physical Review E 94(5) (2016), 053102.
[17] Yu, R., Bai, X.-S., Bychkov, V., (2015) Physical Review E 92 (6), art. no. 063028.
[18] V. A. Sabelnikov and A. N. Lipatnikov (2017), Annu. Review of Fluid Mech. 49, 91-117.
[19] Paul F. Fischer, James W. Lottes and Stefan G. Kerkemeier (2008) http://nek5000.mcs.anl.gov