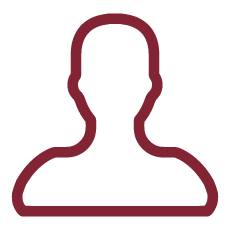
The detection of continuous waves (CWs), which are gravitational waves emitted by non-symmetric rotating neutron stars, is one of the missing pieces in gravitational wave astronomy. In our Galaxy, billions of neutron stars are expected to be present, although only a few thousand have been detected, mainly pulsars, through their electromagnetic radiation.
The information provided by electromagnetic observations is crucial to constrain the signal parameter space, lower the computational cost of a CW search and increase the number of potential targets. Depending on the available information about the source, different searches can be set up. When the only known information is the source sky position the optimal choice is to perform a so-called directed search.
The aim of this proposal is to test and improve the performances and study the results of a new directed search pipeline developed within a new data analysis framework called Band-Sampled-Data, pointing to real astrophysical objects chosen from the last release of the IBIS-INTEGRAL and the Fermi-LAT catalogs. The search will be applied to interferometric data from the LIGO and Virgo detectors, which third observational run (O3) is planned to start with an enhanced sensitivity in Fall 2018. Given the good chances to detect a CW in O3 data, an important step is to understand which physical properties of NSs internal composition (such as the equation of state) can be inferred from the gravitational wave signal.
The detection of CW signals is one of the missing pieces in gravitational wave astronomy. In particular, the detection of signals emitted by isolated neutron star can help to understand better the high-density conditions of matter in those fascinating compact objects.
Indeed, with a CW detection, it is possible to infer the degree of asymmetry in the star, called ellipticity.
The star ellipticity can provide some hints of the internal composition of a NS, since it encodes the information on the maximal deformation that a neutron star can sustain, hence hints of the star equation of state.
Typical values given by theoretical expectations place a maximum ellipticity at 10^(-5) - 10^(-3) depending on the star equation of state. The last CW searches using LIGO-Virgo data placed even stringent constraints.
A detection of stars with ellipticities higher than the predicted theoretical values, which is valid for NS composed by normal matter, will strongly suggest the existence of NS composed by exotic matter, such as strange quark stars or hybrid ones.
In general, the commonly accepted model of NS emission which is spinning down is that of the magnetic dipole radiation (MDR). For this model the braking index is n=3, although observations of isolated pulsars provide values of n in the range 1 In a detection perspective, it is important to understand the connections between the macroscopic parameters that can be measured with EM counterparts (generally Mass and Radius) and the parameters that can be inferred from the GW signal such as the ellipticity and the braking index. In particular, from the GW signals with an EM counterpart (with measured rotational period and period derivative), it is possible to discriminate between the main cause of the asymmetry of the star: the presence of crust deformations ('mountains'), strong internal magnetic fields or others, looking at the proportionality between the spin frequency and the GW emitted frequency. The detection of signals from NS will improve our knowledge about NS galactic population, for example, the detection of CWs from the Galactic center region can explain the not-understand origin of the GeV excess measured by Fermi, as a radiation emitted by a population of unresolved pulsars shield by the presence of interstellar medium ('missing pulsar problem') [Bartels et al., Phys. Rev. Lett., 116 (2016)]. Finally, given the extreme nature of those objects, it will be possible to probe General Relativity (and electromagnetism) in its strong regime. In case of no detection, we should start asking if the model used to describe the emission mechanism is accurate enough or if there is the need to consider more deviations from it or even more exotic mechanisms. The study of neutron star interior composition is a challenging problem which involves several disciplines spanning from astrophysics, nuclear physics, and gravitational wave astronomy.
These relativistic stars also represent a unique laboratory where to test strong and weak interaction in regimes that can not be reproduced on Earth.