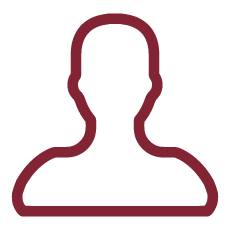
Recently, decades of theoretical and experimental work on gravitational waves (GWs) physics have finally paid off in the historical detections of signals emitted by comparable mass binaries of black holes (BH) and neutron stars (NS) made by the LIGO/Virgo collaboration. This has opened up the possibility of GW astronomy, which will certainly continue to grow and open new paths to explore fundamental physics in the following decades.
To expand this even further, new GW observatories are already planned for the future, namely the space-based observatory LISA, which will allow us to explore different sources than the LIGO/Virgo detectors, such as supermassive black holes in extreme-mass ratio inspirals (EMRI). These will give us new insights both into the nature of these objects that are commonly found in the center of galaxies and into the very foundations of General Relativity (GR) and its possible modifications.
However, for this to come to be we must pave the way for this new experiment, much as it was done before the LIGO detections. EMRIs are remarkably different systems from the comparable mass binaries routinely observed by LIGO/Virgo detectors. Building tailor-made GW waveform models is therefore essential to search for new physics with EMRIs. With this project, we propose to investigate the nature of BHs and other extremely compact objects in the context of EMRIs by studying their gravitational deformations that can be found encoded in their quadrupole, which summarizes the details of its internal structure. Quadrupolar effects may leave a footprint in the GW emitted during the inspiral, which can be measured by LISA with exquisite precision, providing invaluable information on the physics of the binary. These must be considered when building the aforementioned models, so, ultimately, our project aims at giving a contribution to the modelling of EMRIs, which is part of the research lines of several international groups.
The recent detections by the LIGO/VIRGO collaboration have shown that the project of using GWs to do astronomy is feasible and will certainly lead to promising results in coming years. To do so, however, it is necessary to prepare the theory and models to be used by future, more sensitive detectors, such as the LISA interferometer. As such, both facets of our project are extremely timely, as knowledge of EMRIs is still lacking when compared to the knowledge of comparable-mass binaries built for the current detectors.
While recent LIGO/VIRGO data from BHBH binaries points to the expected result of a zero Love number for spinning black holes, the error associated to this measurement is still too large for definite conclusions. For the EMRIs that will be detected by the LISA experiment, on the other hand, a central black hole with a nonzero Love number is expected to have a big impact on the detected waveform leading to constraints that could be down to 8 orders of magnitude smaller than the current ones [Pani & Maselli, arXiv:1905.03947]. As such, theoretically computing the Love number of a spinning BH before data from LISA starts streaming is undoubtedly relevant. Given the aforementioned precision, a small but nonzero Love number will be crucial to correctly model the waveform and extract it from the detection. On the other hand, confirming that the Love number is zero will turn a detection of a nonzero Love number into a "smoking gun" that the primary of the EMRI in question will not be a BH but will indeed be some supermassive, exotic compact object, opening up an avenue for new physics.
Regarding the quadrupole of the secondary, it is expected to give a smaller contribution to the GW phase than its spin and self-force effects. In particular, while the quadrupole induced by the rotation of the body might be observable in the GW phase, the quadrupole induced by tidal deformations seems negligible [Witzany et al., Class. Quantum Grav. 36 075003, 2019]. However, for a few favorable events, the signal-to-noise ratio might still be high enough to allow for measurements of the secondary quadrupole with LISA [Babak et al., PRD 95 103012, 2017]. Notably, all current estimates are based on either back-of-the-envelope calculations or basic scaling arguments. A complete and general analysis of quadrupolar effects in the GW phase even in the simplest case of circular, equatorial orbits is still missing.
Assessing the impact of the quadrupole on the GW phase is relevant because it will give precise information on the accuracy needed to model finite-size terms in EMRIs. Even a tiny effect on the phase can add up during thousands of orbits in EMRIs, leading to a sizable secular effect. If one shows that the secondary quadrupole is indeed negligible, it will be possible to focus theoretical efforts to other relevant terms (like the spin of the secondary). Conversely, if an in-depth analysis reveals that the quadrupole is instead relevant to a certain extent, it will be necessary to model this effect with the needed precision. In this case, the secondary quadrupole might provide an novel window to investigate the equation of states of neutron stars. Either way, studying the quadrupole of the secondary in EMRIs is undoubtedly worthwhile and timely.
Finally, the impact of the quadrupoles of both primary and secondary can be considered together to give an increasingly full picture of an EMRI and of its emission of GWs. This is essential for the building of increasingly accurate waveform templates and, consequently, for taking full profit out of the analysis of the data LISA will provide. Therefore, the results of our project will prove to be of direct use to the community involved in this task of the LISA mission.