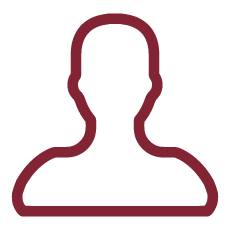
This research project deals with a thorough investigation of new advanced radiating structures for high-frequency wireless power transfer (WPT), relevant to the use of mobile and wearable devices for modern electronic and communication systems. The main unifying idea of the proposal is related to the exploitation of a class of electromagnetic fields, known as leaky waves, that can be employed as a highly efficient instrument to model and synthesize radiating devices having different functionalities. Innovative possibilities of focusing and shaping the electromagnetic fields in the far-field (Fraunhofer) as well as in the near-field (Fresnel) radiative regions are illustrated. A class of planar radiating elements is shown to possess several attractive practical features in terms of power beaming, such as flexibility of the design procedures and simplicity of the geometries. The versatility in achieving reconfigurable radiation patterns (by properly operating on the synthesis of frequency-selective surfaces of the structures) and the capability of limiting undesired diffraction effects (via near-field control) are important distinctive features of the proposed solutions. In the search for adequate wideband configurations, specific attention is devoted to the appealing upper part of microwave spectrum, represented by the millimeter-wave range, particularly useful in the frame of 5-G wireless systems: in such cases, challenging scenarios also arise when the sources perform WPT and simultaneous wireless communication. The strategies for efficiently synthesizing both reconfigurable and limited-diffraction radiating devices will be demonstrated on the basis of a rigorous and powerful theoretical modeling, corroborated by ad-hoc numerical implementations and advanced experimental testing procedures. The fundamental technological achievements of the proposed study are expected to provide a breakthrough for future stimulating operative scenarios involving WPT applications.
As emphasized in the introducing frame, the interest for WPT is tremendously growing in recent times for an increasing number of technological applications. Referring to the key issues related to the energy transmitting/receiving devices, in addition to relatively assessed solutions typically based on 'low-frequency' (MHz) near-field coupling of reactive type (inductive coils etc.), a clear cutting-edge challenge is represented by the use of radiative fields (both in Fresnel and Fraunhofer regions) exploiting the 'high-frequency' ranges of EM spectrum (i.e., microwaves and millimeter waves).
Even though a number of studies is present in the literature for the canonical microwave ranges (up to few GHz), the employment of higher frequencies, i.e., millimeter waves up to terahertz radiation, has not been intensively studied until now for WPT application, mainly due to the inherent path losses: nevertheless, this topic is currently under investigation for various reasons, as it can involve the future EM spectrum for the development of 5G communication or, from another aspect, it may allow for more compact and focusing NF devices. The exploitation of the emerging mm-waves is therefore a big technological challenge with few solutions yet available, but at the same time it looks particularly promising, due to the inherent miniaturization of the devices while preserving the efficiency of the wireless systems.
This project proposes unexplored and versatile design strategies for radiating systems aimed at HF WPT applications. As already introduced, one fundamental innovative distinctive feature of the proposed research is represented by the unifying theoretical approach, where the leaky-wave concept offers a powerful liaison for quite different operational strategies involving WPT. A fundamental advantage of this theory is represented by the fact that it allows for gaining a remarkable physical insight into a variety of significant EM wave phenomena with affordable mathematical complexity. This also enables us to outline very efficient and accurate methods of analysis and synthesis of relevant devices.
In addition to the desirable characteristics of methodological nature, the solutions of LW radiators proposed in this research present unprecedented attractive features in the context of WPT, which furnish significant potential advances in the state of the art of this applicative sector, e.g.:
- the capability of designing similar geometries of planar radiating elements, both for MW and mm-waves, based on the working principles of partially open Fabry-Perot cavity structures where a leaky wave can strongly dominate the propagation and radiation properties;
- the simplicity of the feeding structure, which in general can be obtained by coupling elementary sources (such as coaxial probes or slot dipoles), thus avoiding complex and costly networks typical of standard array configurations;
- the versatility in the reconfiguration of the beam features, related to the control of the aperture field that can be associated to a proper design of the partial reflecting surface of the structure;
- the possibility of adding relatively-simple physical degrees of freedom for shaping the radiation pattern both in far-field and in near-field, allowing in this case also for a significant control of the undesired diffractive and dispersive phenomena;
- the overall inexpensive nature of the envisaged radiating solutions, related both to straightforward efficient methods of analysis and synthesis and to the notable simplicity of the proposed physical structures.
The specific innovative features of the proposed class of radiators can be summarized through different aspects:
- the capability of continuously transmitting power to different devices, even if they move, thanks to the reconfigurable properties of LWA and the communication of the mobile Rx positions to the fixed Tx;
- the powerful design of cost-effective Tx antennas exploiting the advantages of low-cost technology;
- the possibility of focusing EM energy in the radiative NF at mm-waves by means of beams launched by original LW radiators able to control diffraction and also dispersion when operating on adequately wide bandwidths.
Preliminary studies on these topics, recently developed by the proponent group (see, e.g., some of the listed References in this project), have emphasized the feasibility of such innovative solutions for radiating elements in different areas of application. The distinctive features of the suggested structures look particularly promising for cutting-edge WPT applications, involving both static/dynamic FF fixed-frequency pencil or scannable beams and also limited-diffracting NF beams. It can be reasonably expected that the outcomes of this study will provide future designers with clear and reliable rules for assessing the best strategies to implement versatile WPT links, also accounting for all the environment involved.