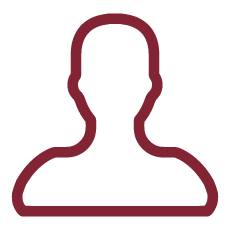
Gas hydrates consist of hydrogen-bonded water frameworks enclosing guest gas molecules and have been the focus of intense research for almost 40 years, both for their fundamental role in the understanding of hydrophobic interactions and for gas storage and energy-related applications. This research project is focused on the water-hydrogen compound (hydrogen hydrate). Hydrogen hydrates can trap hydrogen at mass fractions competing with those of the best materials presently used for hydrogen storage, having the advantage of being environmentally friendly. Pressure is a key parameter in the study of hydrogen hydrates as it remarkably increases their temperature range stability and their storage capability, and it induces substantial variations in the water-hydrogen distances as well as structural transitions. The aim of our project is to apply a combination of experimental techniques, including X-ray and neutron diffraction, quasi-elastic neutron scattering, Infrared and Raman spectroscopy, to characterise the structural stability of H2 hydrate structures, their H2 up-taking, and the new H2-rich structures it forms under HP. The outcomes of this project will be also applicable to a more profound understanding of planetary interiors where H2-H2O hydrates under HP are largely present at depths.
The properties of hydrogen hydrate are of fundamental importance in chemical physics and have been the focus of an increasing number of studies in the last years. Since hydrogen molecules are light and because of the nanoscale size of their confinement, hydrogen molecules trapped in hydrate structure inherently constitute quantum-mechanical objects.
As established experts in the field of high pressure, molecular materials and their structural and dynamical characterization, we want to use a combination of experimental methods to characterize water-hydrogen interaction in this material, the p-T range of its stability and its potentiality as H2-storage material.
Hydrogen is looked upon as the next-generation clean-energy carrier; the search for an efficient, operationally convenient and cost-effective material for storing hydrogen reversibly has been, and is being, pursued relentlessly. The use of hydrogen hydrate for hydrogen storage has several advantages, including their environmentally friendly nature, the lack of safety risk due to their non-explosive nature, and the possibility to perform in principle an infinite number of adsorption/release cycles (there is no chemical change of the substance involved) [2]. The low-pressure clathrate phase of hydrogen hydrate contains 3.8 weight % of molecular hydrogen [3], which compares positively with the mass fractions of some of the best materials presently considered for hydrogen storage. Its application for hydrogen storage is hindered by the slow diffusion rate of hydrogen inside its water skeleton. Some phases formed by hydrogen hydrate at higher pressures contain an even higher amount of hydrogen, particularly the so-called C2 structure, which shows an exceptionally high H2:H2O molar ratio of 1 [4, 5]. However, practical application for hydrogen storage is made difficult by the high pressure needed to form these compounds. Considerable research efforts are currently aimed at exploring possibilities for stabilizing hydrogen hydrate at lower pressures. A pragmatic approach to reduce the formation pressure to the range attainable in the industry would be to employ binary hydrates with H2 and a ¿helper¿ molecule such as tetrahydrofuran, whilst curtailing storage capacity [6]. The capability of hydrogen hydrate to represent a mean of storing hydrogen in energy applications received a new boost by the recent discovery of the phase C0 [7, 8, 9]. The C0 phase forms at pressures above 0.4 GPa, has a H2:H2O molar ratio of up to 0.4 (4.5 weight %), and contains spiraling channels where the guest molecules can easily diffuse. In addition, it can be easily recovered at ambient pressure, where it is metastable at temperatures below 120 K [8].
A key focus to demonstrate the viability of hydrogen hydrate for hydrogen storage relies on a detailed molecular-level understanding of the H2 behavior in this system.
The study of hydrogen hydrate at very high pressures could also reveal some new physical phenomena (orientational ordering of the hydrogen molecules, hydrogen bond symmetrization of the water network, pressure-induced amorphization) [10, 11].
Finally, the study of the phase diagram of hydrogen hydrate is also of interest in planetary astronomy. Since hydrogen and water ice are common constituents of the Universe, it is very likely that under the right circumstances hydrogen hydrate will be formed. This could occur in icy moons for example [2] or icy giants like Saturn and Neptune. Hydrogen clathrate hydrate is indeed likely to be formed in the high-pressure nebulae that formed the gas giants of the solar system [12].
Our team will use HP to provide a breakthrough in the understanding and exploitation of storing capability and HP-enhanced stability of hydrogen hydrate.
[20] Ranieri, U. et al. Nature Comm. 2017, 8, 1076 ; [21] Van Kranendonk J. Solid Hydrogen Springer 1983; [22] Getman, R. B. et al Chem. Rev. 2012, 112; [23] R.M. Herman, et al. Physica B 404 (2009) 1581 ; [23] Hirai, et al. J. Phys. Chem. B 2002, 106, 11089; [24] Sanloup, C., et al. PNAS USA 2002, 99, 25; [25] Hirai, et al. J. Chem. Phys. 2010, 133, 124511; [26] Schaack, S. et al. PNAS 2019,116, 162049; [27] Schaack, S. et al., J. Phys. Chem. C 2018, 122, 11159; [27] Machida, S.; et al. J. Phys. Chem. Solids 2010, 71, 1324; [28] Machida, S.-i.; et al., Phys. Rev. B 2011, 83, 144101; [29] Dyadin, Y. A.; et al. Mend. Comm. 1999, 9, 209 ; [30] Kadobayashi, H.; et al.. J. Chem. Phys. 2018, 148, 164503; [31] Dubrovinsky, L.;et al. Nature 2015, 525, 226; [32] Klotz, S.; Besson, J. M. Appl. Phys. Lett. 1995, 66, 1735; [33] Boehler, R.; et al. Rev.Sci.Instr. 2017, 88, 083905; [34] Boehler, R.; et al. High Pressure Research 2013, 33, 546; [35] Bove, L. E., Phys. Rev. Lett. 2013, 111, 185901; [36] Komatsu, K.; et al. High Pressure Research 2014, 34, 494.