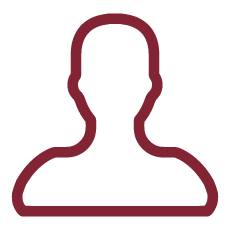
We propose a novel experimental approach to address the role of defects on the electronic and transport properties in graphene. To this aim we will use resonant Raman spectroscopy with excitation in the infrared and compare experimental data to ab-initio theoretical calculations. Raman scattering is a spectroscopic technique based on inelastic scattering of light, and provides information on vibrational and other low-frequency modes in a physical system. Resonant Raman spectroscopy, involving electronic transitions between real states in the scattering process, yields information also on electron-phonon (e-ph) and electron-electron (e-e) interaction processes. Noteworthy, while non-resonant Raman cross sections are proportional to the fourth power of the excitation laser energy, in a resonant process the scattering intensity can be greatly enhanced independently on the exciting wavelength. In this context, the introduction of infrared (IR) lasers instead of visible ones will allow the study of the interplay between defects, electronic and thermal transport in graphene with an unprecedented accuracy. Indeed, Raman spectra obtained with excitation laser wavelength of 1 micron and longer may yield a different peak lineshape. These vibrational modes, in particular their relative intensity and lineshape, can now be modelled starting from first-principle atomistic calculations based on the density functional theory (DFT) providing an extremely carefull test for the most advanced electron-phonon scattering theories. We will artificially induced low density of defects in graphene with electron-beam and follow the modification of the Raman spectra, comparing it to theoretical calculations.
Structural defects break the symmetry of the carbon lattice, significantly altering its properties. On the one hand, the excellent electrical and mechanical properties of graphene are substantially lowered by introducing defects. On the other hand, the sensitivity of graphene-based chemical gas sensors and the catalytic reaction can be enhanced by the adsorption energy reduction at the defect sites [1,2,3,4,5,6] Moreover, a theoretical study suggested that the defects at the polycrystalline boundary of graphene can create a tunable transport gap [7], which is essential for graphene nanoelectronic applications.
Most of the experimental effort to study defects in graphene has been devoted to the study of defects induced in graphene either for fluorination and mild oxidation or of ion-bombardment [8,9,10], but some effort has also been devoted to study the effect of of electron beam irradiation [11,12]. Within this project we aim ad addressing low density of defects, exploiting the higher sensitivity that we expect to have with an excitation laser in the infrared, assessing their role in the transport properties.
The analysis of the resonant Raman peaks for low energy excitation energies could provide an efficient tool to address small amounts of defects not only in graphene flakes, but even in 2D-encapsulated graphene flakes with unprecedented accuracy: indeed, the D' mode should be clearly visible, because of its resonant nature at variance with the G peak [13], thus allowing for a better determination of its intensity and linewidth. Moreover, the D mode should be clearly visible also for hBN-encapsulated graphene, as no signal from the hBN 1370 cm-1 LO phonon mode (close in energy to graphene D mode) is expected. As an added feature, the excitation energy can be chosen to be well below the gap for two-dimensional transition-metal dichalchogenides (2D-TMDs), avoiding any absorption from the above encapsulating layers, yielding thus a high-throughput, accurate Raman characterization of the graphene flakes.
Moreover, ab-initio calculations have identified the shape of 2D Raman peak in three and four layers graphene with either Bernal or rhombohedral graphene, or a combination of the two [14]. By tuning the excitation energy down to, and beyond, the band gaps one could further increase differences in the 2D peak lineshape and intensity among different graphene stacking modes. This experimental approach could be an efficient, non-destructive way to identify the precise stacking sequence in a multilayer sample with a space lateral resolution of a few microns.
[1] Y.H. Zhang et al. Nanotechnol. 20, 185504 (2009).
[2] F. Schedin et al. Nature Mater. 6, 652 (2007).
[3] W.Yuan and G. Shi, J. Mater. Chem. A. 1, 10078 (2013).
[4] D.H. Lee et al. Phys. Rev. Lett. 106, 175502 (2011).
[5] D.J. Li et al. Nano Lett. 14, 1228 (2014).
[6] G.Y. Lee et al. J. Mater. Chem. A. 5, 1941 (2017).
[7] O.V. Yazyev and S.G. Louie Nature Mater. 9, 806¿9 (2010).
[8] A. Eckmann et al., Nano Lett. 12, 3925 (2012)
[9] A Eckmann, et al., Physical Review B 88 (3), 035426
[10] M.K. Daniels et al, Journal of The Electrochemical Society 162(4):E37 (2015)
[11] X. Yu et al, Scientific Reports 5:12014 (2015).
[12] D. Teweldebrhan and A.A. Balandin, Appl. Phys. Lett. 94 (1), 013101 (2009)
[13] L. G. Cançado, A. Jorio, and M. A Pimenta, Phys. Rev. B 76, 064304 (2007).
[14] A. Torche, F. Mauri, C. Charlier and M. Calandra Phys. Rev. Materials 1, 041001(R) (2017)