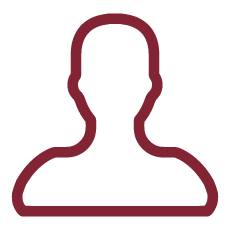
Due to their desirable properties such as high ionic conductivity, negligible flammability and tunability, ionic liquids (ILs) have been recognized as promising electrolyte materials in lithium-ion batteries with improved safety. In ILES project non-commercial ILs, formed by ether-functionalized N-ethoxyethyl-N-methyl piperidinium (P1,2O2) cations and bis(fluorosulfonyl)imide (FSI) or difluoro(oxalato)borate (DFOB) anions, will be synthesized and investigated. These ILs, combined with a suitable lithium salt, were recently proposed as electrolyte components in Li cells, due to their low crystallization attitude and improved ionic conductivity. Anyhow, important features, related to their stability and effectiveness for long-term safe applications under an extended temperature range, were not fully assessed. Here, temperature-activated physical and chemical processes, such as degradation and phase transitions, will be explored in both pure ILs and their mixtures with a lithium salt. The effect of different Li+ sources and of their concentration in the ILs will be considered ranging from widely used salts (i.e., LiPF6) to tailored LiFSI and LiDFOB. Moreover, the addition of molecular aprotic solvents, such as dimethyl carbonate and ethylene carbonate, adopted in commercial battery electrolytes, will be investigated with the aim of enhancing ions dissociation and mobility in the IL-based mixtures. The resulting novel electrolyte compositions will be characterized in terms of physico-chemical properties related to their thermal stability (vaporization, decomposition, melting, glass transition, flash point) and of electrochemical performances (ionic conductivity, electrochemical stability window, interfacial resistivity and charge-discharge capacity in conventional battery configurations). Overall, ILES proposes an original, very appealing approach to correlate fundamental thermodynamic properties of IL materials to their applicability in energy storage batteries.
With an increase in the energy density required to be supplied by batteries, there is growing demand for improving their reliability. While electrodes determine the nominal capacity of batteries, electrolytes act as a critical component in realizing the safety specifications. Electrolytes are generally prepared by dissolving lithium salts in aprotic solvents that have a high dielectric constant. 1M LiPF6 dissolved in ethylene carbonate and dimethyl carbonate is one of the benchmark electrolytes for lithium ion batteries [1]. However, carbonates possess a high vapor pressure and amplify the safety issues. Thus, the replacement of carbonate solutions with nonflammable materials is strongly recommended [2].
There are two major families of alternative electrolytes: one is solid electrolytes and the other is ionic liquid (IL)-based electrolytes. Solid electrolytes such as inorganic ceramics [3], organic plastic salts [4], and dry polymer electrolytes [5] have negligible flammability and an ability to prevent leakage of electrolytes. However, these compounds have drawbacks in realizing a high ionic conductivity and a good electrode-electrolyte interfaces with small charge transfer resistances, due to their crystallinity.
In contrast to this, ILs are recognized to be potential alternatives with high ionic conductivity and excellent thermal stability [6]. ILs are molten salts composed only of ions. They exhibit negligible vapor pressures because of coulombic interactions, which are weak enough to keep them liquid and to enhance desirable ionic conductivity [7].
Generally, ILs are used in designing electrolytes in three ways: i) as an electrolyte itself (IL-Li salt system), ii) as a main component in electrolytes (solution-in-IL), or iii) as an additive for electrolytes (IL-in-solution). Increased IL concentration strongly reduces self-extinguishing time of electrolytes [2]. However, the drawback of the use of ILs as a principal component is increased overpotential of cells due to high internal resistance related to viscosity. To investigate and address these two opposing properties, IL-Li salt systems and solution-in-IL electrolytes will be compared in the proposed ILES project.
Among various anions, to form suitable ILs, bis(trifluoromethanesulfonyl)imide ([TFSI]) andPF6are the most widely used for battery applications. In addition to these, FSI anions have also strong potentiality. The ILs consisting of FSI anions have low viscosity and high ionic conductivity, although FSI anions have a small ion size and high charge density. This is because the negative charge on FSI anions is delocalized by the fluorine atoms. Moreover, the ILs containing FSI anions are known to form solid electrolyte interphases (SEI) at low voltages, which allow a sufficient charge transfer of lithium and protect both the electrolyte itself and electrode from decompositions [8]. At the same time, our choice of ILs containing difluoro(oxalato)borate (DFOB) anion, is due to the capability to form protective layers on both positive, high voltage cathodes and graphite-based anodes [9].
To suppress the crystallizing tendency of both FSI and DFOB anions, they must be combined with cations having a delocalized charge or flexible substituents. As an alternative to unsaturated bonds, the functionalization of the aliphatic cations by using alkoxy groups has been found to be effective [10].
Taking these facts into account, N-ethoxyethyl-Nmethyl piperidinium FSI and N-ethoxyethyl-N-methyl piperidinium DFOB and their mixtures with Li-salts and carbonate solvents, will be originally investigated in this project, with the ambitious goal of correlating their thermal and electrochemical properties.
[1] T. Kawamura, S. Okada, J. Yamaki, J. Power Sources 156 (2006) 547-554
[2] A. Guerfi, M. Dontigny, P. Charest, M. Petitclerc, M. Lagace, A. Vijh, K. Zaghib, J. Power Sources 195 (2010) 845-852
[3] Y. Aihara, S. Ito, R. Omoda, T. Yamada, S. Fujiki, T. Watanabe, Y. Park, S. Doo, Front. Energy Res. 4 (2016) 1-8
[4] D.R. MacFarlane, M. Forsyth, Adv. Mater. 13 (2001) 957-966
[5] R.C. Agrawal, G.P. Pandey, J. Phys. D Appl. Phys. 41 (2008) 223001-223018
[6] M. Armand, F. Endres, D.R. MacFarlane, H. Ohno, B. Scrosati, Nat. Mater. 8 (2009) 621-629
[7] M. Galinski, A. Lewandowski, I. Stepniak, Electrochim. Acta 51 (2006) 5567-5580
[8] R. Kerr, D. Mazouzi, M. Eftekharnia, B. Lestriez, N. Dupre, M. Forsyth, D. Guyomard, P.C. Howlett, ACS Energy Lett. 2 (2017) 1804-1809
[9] M. Xu, L. Zhou, L. Hao, L. Xing, W. Li, B.L. Lucht, J. Power Sources 196 (2011) 6794-6801
[10] J. Reiter, E. Paillard, L. Grande, M. Winter, S. Passerini, Electrochim. Acta 91(2013) 101-107