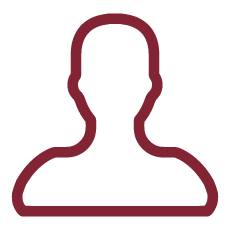
Chiral objects do not possess mirror symmetry; they exist in the ¿left¿ and ¿right¿ version, and come in all dimensions, from DNA to chiral galaxies. Chirality governs the interaction of the object with the outside world, defining the properties from molecular toxicity and biological activity, to new degrees of freedom in photonics. Chiral nanostructures are a hot-topic in photonics, as they are able to control the chiro-optical response at the nanoscale. They are designed to differently interact with left and right circularly polarized light (LCP and RCP, respectively); this difference is usually defined as circular dichroism (CD), and can be detected as the differential absorption between LCP and RCP excitations. On the other hand, chiral nanostructures, coupled with emitting layers, can possess CD in the emission, i.e. they can emit light with high degree of circular polarization.
In this proposal we want to experimentally study chiral low-cost metamaterial, coupled with emitting material, for the tunable CD in emission in the visible and near-infrared range. We have previously designed and experimentally studied low-cost chiral plasmonic metamaterials, fabricated in collaboration with the University of Padova (NanoStructures Group); specifically, we characterized chirality in absorption, extinction, and diffraction in the Nonlinear Photonics laboratory at the SBAI Department. Very recently, we numerically proposed chiral emission from these nanostructures by coupling them with emitters. In the present proposal, we optimize design of plasmonic low-cost metasurfaces for the coupling with industrially relevant dye molecules, and fabricate them in Padova. Finally, at the SBAI Department, we construct a new imaging set-up, which allows for the chiral excitation of the nanostructures, and chiral detection of the emission from the coupled system. The ultimate goal of this project is the experimental observation of tunable chiral emission from low-cost metasurfaces.
Chiral nanostructures are usually fabricated by expensive and slow EBL technique, while the additional coupling with the emitting material increases the overall complexity and unpredictability of the design. Instead, our NSL approach with tilted plasmonic deposition allows for fabrication of low-cost 3D or 2D asymmetric metasurfaces. The 3D metasurfaces consist of asymmetrical plasmonic shells on the top of 2D array of dielectric PSN, while the 2D metasurfaces are based on the ENHA (after the PSN removal). Our fabrication, in collaboration with the Padova group, offers low-cost, self-assembled samples of high quality; we previously measured chiral absorption in such samples by means of photo-acoustic technique, extinction spectroscopy, and photo-deflection technique. Our recent numerical investigation opened the perspective to couple the metasurfaces with thin layers of emitting materials. Specifically, the metasurfaces need to be embedded in the dielectric, with a layer of emitting material above the plasmonic layer. While the 3D Au-based metasurfaces are suitable for chiral emission in the near-infrared (i.e. for chiral emission of Er3+ molecules), the 2D metasurfaces can work in the visible if the plasmonic layer is Ag (i.e. for chiral emission of Lumogen Red dye). Working in these spectral ranges places the starting diameter of the PSN in the range of 350-550 nm.
We start with a numerical method which provides the average circular polarization degree on the far-field hemisphere, emitted from the 3D and 2D metasurfaces coupled with quantum emitters. We investigate the emission from large ensembles of randomly oriented, uncoherent electric dipole sources, which are homogeneously embedded in a dielectric above the plasmonic layer. The geometric parameters of the 3D and 2D metasurfaces, as well as their fabrication margins, are based on our vast previous experience in modelling of the NSL-based samples. Once the emission and excitation wavelengths are known, the metasurface is numerically optimized to exhibit two parallel phenomena: chiral emission, and its tunability at the excitation wavelength. In the 3D metasurface, localized surface plasmons of the plasmonic nanoshell couple with in-plane Rayleigh scattering resonances, which leads to chiral surface lattice resonances. We previously showed that these resonances lead to the high electromagnetic field confinement over the refractive index matching layer (J. Phys. Chem. C 2019, 123, 38, 23620¿23627). They can appear in two different spectral regions, thus overlapping with the emission and excitation wavelength. At the Er3+ emission wavelength, the nanoshell chirality leads to the breaking of the symmetry in the emitted modes, with preferential LCP or RCP emitted in the opposite directions of the far-field hemisphere. At the Er3+ excitation wavelength, the surface lattice resonances can enhance the absorption and tune the intensity of the emission. Physics of the 2D metasurface is different, as the behavior of plasmonic ENHA is governed by surface plasmon polaritons. We previously showed that the ENHA offer two different spectral regions of opposite chirality (Opt. Quantum Electron. 2020, 52, 176). We therefore overlap the high energy chiral region with the Lumogen Red excitation (around 532 nm), while the low energy ENHA chirality governs the Lumogen Red F emission (around 615 nm).
NSL offers many degrees of freedom for the shapes and dimensions of the ENHA and plasmonic nanoshells. Next, we provide the Padova group with the needed fabrication parameters: polystyrene diamater choice (which defines a), RIE etching time (which defines Dp), and the angles of the metal deposition, which finally define the chirality. Finally, we construct the new experiment based on Fourier back focal plane imaging, which resolves chirality in both excitation and emission. Our laboratory is equipped with the widely tunable near-infrared laser (Chameleon Ultra II by Coherent Inc), which allows for the fundamental wavelength range from 680nm to 1080nm. The second harmonic of this laser, decreased in intensity by additional components, allows for the excitation of the metasurface emission; a pair of the linear polarizer (LP) and quarter waveplate (QWP), tunes the excitation polarization. Next, we catch the high part of the emitted light by a high numerical aperture objective, for the investigation of the highest possible angular far-field cone. We place the second (LP,QWP) pair working in the emission range, and project the polarization-resolved emission to the set of lenses, which together form a typical 4f system. After passing through this system, the emitted k-space image is formed on a CCD camera. To the best of our knowledge, these spectrally rich chiral phenomena have not been experimentally investigated before.