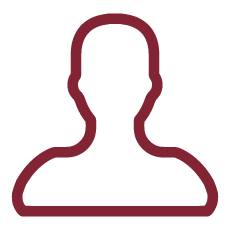
Bioprinting is a tool increasingly used in tissue engineering laboratories. As an extension to classic tissue engineering, it enables high levels of control over the spatial deposition of cells, materials, and bioactive factors. Extrusion bioprinting is the most commonly used technique, where high viscoelastic solutions of materials and cells (bioink) are required to ensure flow through the nozzle and good shape fidelity of the printed tissue construct. However, the encapsulated cells are often restricted in spreading and proliferation by dense biomaterial networks originated from the gelation of bioinks. Bioprinting macroporous hydrogel fibres can handle such a problem by allowing for adequate cell motility and their organization into functional tissues. This project aims to develop aqueous two-phase emulsions where the dispersed phase droplets act as the pores templates, and the continuous phase is photocrosslinked, leading to predesigned cell-laden hydrogel constructs. Such a fully aqueous environment would ensure appropriate mass exchange and good cell survival.
Rotational rheometry is routinely used to assess ink printability and as a guide to ink formulation. However, the flow imposed by currently available instrumentation is not representative of the bioink flow pattern inside an extrusion system, making difficult the correlation between results and bioink printability. Furthermore, rotational rheometry does not allow predicting the effect of extensional and shear stresses at the syringe-nozzle junction and inside the nozzle, respectively, on cell viability. Recent advances in microfabrication and microfluidics have spurred the development of microfluidic viscometers. These instruments require low sample volumes, favouring systematic investigation and generate a flow field replicating that experienced by the bioink during extrusion. These features make microfluidic viscometry a tool particularly suited in the screening of bioink candidates.
The proponent of this project is the docent of ¿Laboratorio di Biopolimeri e Biomateriali¿, a course encompassing a relevant part of teaching labs, including, among the others, the rheological characterisation of polymeric solutions and the determination, through viscometric measurements, of the molecular weight of representative biopolymers. The instrumentation required is meant to replace a capillary viscometer and a rotational rheometer. Both are obsolete instruments, exclusively used for teaching purposes. Therefore, one of the motivations underlying the present request is to replace such instrumentation to maintain high-quality education and training standards and provide the students with an updated view of modern techniques used to characterise complex fluids.
From a research perspective, microfluidic viscometry offer new opportunities, complementing classical rheometry, in the characterisation and flow behaviour of manufactured and naturally occurring biofluids, currently a relevant and active research field from basic and applied standpoints. Steady shear viscosity is undoubtedly the most widely characterised material property of these fluids. Although widely adopted, macroscale rheometers are limited by sample volumes, access to high shear rates, hydrodynamic instabilities, and interfacial artefacts. The limits of classical rheometry are discussed in some detail to highlight the benefits of microfluidic viscometry.
-Low viscosity samples. Measurement of shear rheology over a broad range of shear rates for water-like fluids such as dilute macromolecular solutions, bacterial fluids, human body fluids is challenging with macroscale rheometry. The limitation arises due to the lowest torque that can be reliably measured. This particularly affects the acquisition of shear viscosity data at low shear rates. Data can be potentially acquired at higher shear rates, but then secondary flow and inertial instabilities might kick in, limiting the range of reliable data. Microfluidic viscometer can reliably measure viscosities as low as 0.001 Pas×sec in a shear rate range between 1 ¿ 10E6 sec-1.
-Small sample volumes. The amount of sample available for most synthetic complex fluids is large enough that standard rheometric methods can be used for shear rheology characterisation. However, this is not the case for many biofluids, including monoclonal antibody solutions, DNA solutions, saliva, and exudates. Fluid volumes can also be a limitation when a diverse set of assays must be conducted with the same sample. Thus, macroscale rheometers are incapable of measuring the rheology of microliter sample volumes. Microfluidic viscometers benefit from consuming less sample, which is especially important for biofluids.
-Inertial and secondary flows. The viscometric flows that are employed in macroscale rheometry are prone to inertial instabilities and secondary flows. As a result, it is challenging to obtain shear viscosity data at high shear rates (>1.000 s-1). However, viscosity data at very high shear rates are essential for various applications, including lubricants, inkjet and extrusion printing. Microfluidic devices allow obtaining viscosity data at high shear rates without crossing over to turbulent regimes. This because the Reynold number is typically small in microfluidic flows since it depends on the smallest length scale of flow (i.e. channel height), indicating that viscous forces dominate over inertial forces. Although the Reynolds number is small, very high shear rates can be achieved since the characteristic length scale is of the order of microns. Furthermore, microfluidic devices provided with a contraction offer the opportunity to study the extensional flow of highly elastic fluids employed in industrial processes, lubrication, bioprinting.
-Interfacial artefacts. A free interface is inherently present when the sample is placed in a cone and plate, Couette and parallel-plate rheometers. The presence of this interface can produce artefacts in the rheology data. The artefacts arise due to surface tension forces, evaporation of sample, and film formation by surface-active species. These artefacts are particularly problematic with low viscosity fluids, which can mask the fluid¿s true shear rheology. Microfluidic viscometers are closed systems and do not present free surfaces.
-Small-gap errors. In some instances, there is a need to conduct rheometry in small gaps, for example, to minimise sample volume, study confinement effects on rheological behaviour, or achieve high shear rates. In such cases, parallel-plate geometries are convenient to use. However, it is difficult to ensure uniformity of the gap so that artefacts can be avoided. Advances in microfabrication methods have provided opportunities to construct micrometre-scale channels at a length scale compatible with the mesoscale structure of complex fluids allowing for probing their rheology at a wide range of length scales and shear stress.