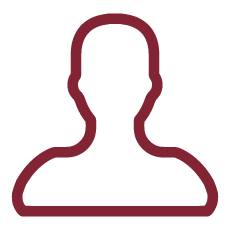
The Human Ether-à-go-go-Related Gene is a gene that codifies for the alpha subunit Kv11.1 of the simply denoted hERG channel. It is a voltage dependent potassium channel found prevalently in the cardiac tissue that contributes to its cell membranes repolarization regulating the heart mechanics. This is possible by the gating mechanism that modulates the transition between the open and closed states of the channel due to the membrane potential variation. Loss of function of hERG channel is associated to the Long QT Syndrome, a condition linked to sudden cardiac death caused by both genetic mutations and assumption of not specific drugs. In order to minimize the risk of producing pro-arrhythmia drugs, the Comprehensive in Vitro Proarrhythmia Assays that allow to study in a computational way this channel to understand whether candidate drugs block it in the closed state or delay its opening. What I propose in this project is to study the hERG channel with a computational approach based on Molecular Dynamics simulations that gives the possibility to explore phenomena with the correct spatial and temporal resolution allowing to connect the protein structure to its function and dynamics. In order to characterize the transition path from open to closed state and vice versa, starting from the solved open structure, at first I will produce a more realistic closed model via Steered Molecular Dynamics simulations and then I will study the gating kinetics by means of Targeted Molecular Dynamics. Finally, I will produce specific mutants of the channel to compare the physiological to the pathological path. Understanding the molecular mechanism underlying hERG channel gating, focusing on the crucial residues and their interactions, is important not only for human health but also to extract the general principles to design artificial nanopores and biosensors with higher selectivity.
This project aims at characterizing molecularly the gating mechanism of the hERG voltage gated channel. It will bring innovations for both biomedical and engineering scientific research. In the first case concerning the production of a new computational closed channel: it will play a key role in many application fields, from bioinformatics to pharmaceutical chemistry. For the first time it will allow to study off-target drug interactions via molecular docking no longer exclusively on the open channel but also on the closed state giving the possibility to identify probable hidden binding sites otherwise not evident observing the open state due to the different protein conformations. Moreover, this realistic model will let to study how the system goes from the open to the closed state elucidating the free energy cost to carry out the transition. The characterization of the gating mechanism with an atomistic resolution via Molecular Dynamics simulations will allow not only to understand the channel physiology but also the molecular determinants of a serious pathology such as the Long QT Syndrome. Besides the kinetics of the process will be the basis for drugs design: characterizing all the states populated by the system during the transition is important to predict the channel conformational changes and consequently all the accessible binding sites to create safer and more effective molecules as future drugs to treat the channelopathy. On the other hand, there will be several advancements in the engineering. Understanding the functioning of an ion channel will inspire the production of artificial biomimetic channels to study various biomolecules in confined spaces and in real time by current measurements. The artificial channels are becoming the focus of attention because, compared with their biological counterparts, they offer greater flexibility in terms of shape and size, robustness and surface properties. Due to their functions, biological ion channels give the possibility to extract the basic principles of selectivity, sensing and regulation related to their molecular composition and to reproduce them artificially depending on the desired application. In fact chemical modification of the interior surface of the artificial channels with functional molecules that closely mimic the biological gating mechanisms may provide a highly efficient way to control and quantify ionic or molecular transport through them in response to ambient stimuli such as applied force, light, pH and specific ions [2]. They have also a rapid and reversible capacity to detect the concentration of confined analytes, for example in a cell, or to identify several analytes concurrently. There are many examples of biomimetic smart channels: the stochastic sensors based on the staphylococcal alfa-hemolysin [3] or the DNA sequencer produced on the transmembrane porin made from DNA origami [4].
In summary, the identification of the hERG channel gating will be useful to reveal the molecular determinants of the Long QT Syndrome in order to design both new specific drugs to treat it and smart artificial channels or sensors with high specificity to an analyte.
References:
[1] Sharan K. Bagal et al., Ion channels as therapeutic targets: a drug discovery perspective. J. Med. Chem. 56, 3, 593-624, 2013.
[2] Xu Hou and Lei Jiang, Learning from Nature: Building Bio-Inspired Smart Nanochannels. ACS Nano, Vol.3 3339-3342, 2009.
[3] H. Bayley and P. S. Cremer, Stochastic sensors inspired by biology. Nature, Vol. 413, 2001.
[4] K. Göpfrich et al., Large-Conductance Transmembrane Porin Made from DNA Origami. ACS Nano, 10, 8207-8214, 2016.