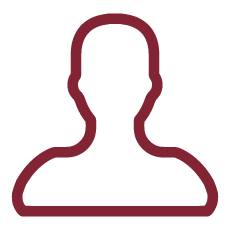
The availability of methods for the accurate characterization of electric and magnetic properties at the nanoscale is a growing request in the field of nanoscience and nanotechnology. Atomic force microscopy (AFM) is a versatile platform to develop Kelvin probe force microscopy (KPFM) for the mapping of work function (WF) and surface electric charges, and magnetic force microscopy (MFM) for the imaging of magnetic fields at the nanoscale which, despite quite widespread, still suffer from severe limitations in terms of quantitative analysis.
In this project we propose to develop KPFM and MFM methods for the accurate quantitative nanocharacterizations. We will compensate for the lack of probes suitable for both methods and for effective multi-materials calibration samples by optimized microfabrication.
As for KPFM, we will focus on optimization of the sensitivity of WF and electric charges measurement, analyzing also the water layer effect with studies in air and vacuum also via correlative probe electron microscopy (CPEM).
As for MFM, we will address the accurate deletion of electrostatic artifacts which limit nanomagnetic quantifications. Tapping mode MFM will be optimized and torsional resonance MFM (TR-MFM) will be developed, a promising technique not yet popular due to the need for magnetical asymmetric tips not commercially available. Then an innovative mehtod combinig KPFM and TR-MFM will be develped for the ultimate deletion of artifacts and maximization of sensitivity to ultralow magnetic fields.
The methods will be used on samples identified as case study with high scientific and technological impact, i.e., polymeric soft colloids known as stimuli-responsive microgels (MGs) and nanoparticles-MGs hybrids with applications from nonlinear optics and soft matter to nanomedicine and materials science and advanced cathodic materials involved in the recycling of end-of-life Li-ion batteries.
The foreseen results of the project would represent a significant advancement in the field of nanometrology. In particular, the developed methods could enable a broad community of AFM users to quantitatively characterize magnetic and electric properties of materials at the nanoscale, using easily accessible and widespread techniques like MFM and KPFM. The developed models will allow a more accurate description of the tip-sample interactions which will be extremely useful not only for researchers directly interested in magnetic and electric nanocharacterization techniques but more in general for the whole community of AFM based nanometrologists. Also, the specifically designed and optimized probes for MFM and KPFM as well as the different calibration samples will represent a major outcome of the project, would represent the basis for specific patents and are potentially highly attractive for producers of AFM instrumentations and equipment. Overall, the methods represented by: (i) techniques, i.e., MFM (in particular the innovative TR-MFM but also TM-MFM), KPFM, and combined KPFM and MFM; (ii) tips; (iii) calibration samples, would represent a tremendous improvement of AFM capabilities for magnetic and electric nanocharacterization techniques. In particular, in the proposers¿ view, a particularly promising technique is TR-MFM in combination with KPFM which was never proposed but has the potentiality to become the most effective tool for electric and magnetic characterizations at the nanoscale allowing one to probe the electric and magnetic field from the sample at unprecedented small distances (a few nm).
Several studies were reported in the last years regarding the regeneration of EoL LIBs electrode materials with the aim to reintroduce them in the LIBs manufactory chain. Cathode materials could be easily regenerated by adding Li source to restore their stoichiometry and then annealed at high temperature [46,47]. Likewise, it was demonstrated that graphite anode material could be reused after its detachment from the copper current collector of EoL LIBs [48]. On the other hand, all the efforts reported in the literature for the regeneration of battery materials involves the manual dismantling of EoL LIBs including separation of cathode and anode from current collectors¿ foil. This way allows for a fine separation of anode and cathode materials and no impurities (e.g. Cu and Al from current collector) are considered. This is a hardly scalable approach to process the huge amount of EoL LIBs that will be produced in the very next few years. The common processes for LIBs recycling include their mechanical pre-treatment producing a fine powder that contains cathodes, anode, fragments of current collectors (Cu, Al) and metallic case (Fe). In addition, differently from the literature reported regeneration methods, the fine powder can contain a mixture of different cathode materials (LiNiO2, LiCoO2, LiNiaMnbCocO2 a+b+c=1) [9]. In order to apply the already developed regeneration procedure, an effective way to easily separate cathodes and anode in the electrode powder, resulting after crushing EoL LIBs, are required. An in-depth characterization of the cathode and anode materials at EoL could pave the way to the development of physical strategies to separate these materials. Magnetic behaviour for nanostructured LiNiO2, LiCoO2, LiNiaMnbCocO2 are not completely understood and, remarkably, data about their magnetic properties at EoL are not reported [11]. Depending on the final state of charge of the batteries when disposed, different lithiation degrees in the cathode materials can be found. Thus the different compositions and crystalline structure of EoL cathode materials can change the magnetic properties and for this reason should be characterized. An in-depth characterization of EoL could enable the magnetic separation of cathode and anode materials and thus closing the loop of LIBs recycling.
Development of electric AFM technique will be valuable for expanding knowledge of soft microgels (MGs). Despite the huge amount of experimental and theoretical works on their behavior, there are still fundamental aspects that remain poorly understood. It has been shown that the peripheral charges have a relevant role in concentrated MGs suspensions [49], as well as in the MGs colloidal stability and in the interaction with other charged colloids [41,42] Recently, it was shown in a combined in silico and experimental investigation that the spatial distribution of the charges influences the swelling behavior of the particles [50]. MGs spatial charge distribution has not been investigated so far by an experiment due to the lack of adequate experimental methods able to determine the spatial charge distribution at single-particle level. The possibility to characterize the charge distribution of MGs at nanoscopic level by electric-AFM would allow an advancement of the knowledge on these systems and their potential applications.