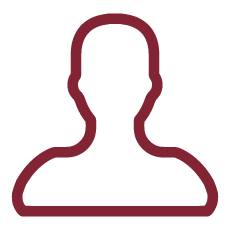
Oligogalacturonides (OGs) are damage-associated molecular patterns (DAMPs) that are recognized by plant receptors as signals of an altered-self and activate the plant immune response. The accumulation of OGs in vivo is favored by the interaction of microbial polygalacturonases (PGs) with plant-derived inhibitors (PGIPs). Transgenic Arabidopsis plants conditionally expressing a chimeric protein made by a fusion between a PG of Fusarium phyllophilum and a plant-derived PGIP, named "OG machine" (OGM), have been generated (1), allowing the release on command of OGs in planta and the consequent activation of defense-related responses. In this way OGs should act as biocontrol molecules to prevent the deleterious effects of one or more challenge of phytopathogenic organisms. If the command persists, plant growth is reduced or completely arrested; this effect reflects the natural trade-off existing between growth potential and plant defense capacity. Notably, a very high level of OGM expression triggers cell death. This project will focus to better understand the molecular mechanism involved in plant defense against pathogens and the key aspects of the trade-off between development and defense that takes place in response to the OGM action. The novel aspect of this project is to improve and monitor agricultural production without the use of pollutants, such as pesticides and fertilizers.
The project combines cellular and molecular biology technique to address fundamental questions that attract many researchers: identify key elements in the regulation in plant defense against pathogens with the aim of improving and monitoring agricultural production without the use of using pollutants, useful for environment and human health. The damage caused to the physical, chemical, biological and social processes has an impact on the reduction of biodiversity. So, to improve the quality of life of the ecosystem, it would be desirable to raise the attention on what could cause irreversible damage, reducing the behaviors that cause alterations and, above all, it would be auspicial to use valid precautionary measures for future generations.
Besides these benefits, the establishment of the ¿-estradiol OGM lines-based analysis represent an important new approach to face this challenge. The new insights obtained in Arabidopsis thaliana plant as a model organism can be applied directly to economically important crop species to increase yield and improve other important agronomic traits.
Publications in high impact, peer reviewed open access journals will complete the results dissemination strategy.
References
1. Benedetti M. et al., Proc. Natl. Acad. Sci. U. S. A. 112, 5533-5538 (2015).
2. Jones J. D. G., Dangl J. L., Nature 444, 323-329 (2006).
3. Boller T., Felix G., Annual Review of Plant Biology 60, 379-406 (2009).
4. Nurnberger T. et al., Immunol.Rev. 198, 249-266 (2004).
5. De Lorenzo G. et al., Trends Immunol 39, 937-950 (2018).
6. Chisholm S. T. et al., Cell 124, 803-814 (2006).
7. Galletti R. et al., Plant Physiol. 148, 1695-1706 (2008).
8. Tsuda K. et al., Plant J. 53, 763-775 (2008).
9. Peng Y. et al., Molecular Plant Microbe Interactions, MPMI06170145CR (2018).
10. Conrath U. et al., Annu Rev Phytopathol 53, 97-119 (2015).
11. Conrath U. . et al., Plant Signal Behav 1, 179-184 (2006).
12. Conrath U. et al., Trends in Plant Science 7, 210-216 (2002).
13. Cote F. et al., Subcell.Biochem. 29, 385-432 (1998).
14. De Lorenzo G. et al., Annual Review of Phytopathology 39, 313-335 (2001).
15. De Lorenzo G. et al., Current Opinion in Plant Biology 5, 295-299 (2002).
16. Galletti R. et al., Plant Physiol. 157, 804-814 (2011).
17. Ferrari S. et al., Plant Physiol. 144, 367-379 (2007).
18. Brutus A. et al., Proc. Natl. Acad. Sci. U. S. A. 107, 9452-9457 (2010).
19. Rodriguez E. et al., FEBS J 283, 1385-1391 (2016).
20. Bruggeman Q. et al., Front Plant Sci 6, 24 (2015).
21. Rioux J. D. et al., Nature 435, 584-589 (2005).
22. Wiermer M. et al., Current Opinion in Plant Biology 8, 383-389 (2005).
23. Abreu M. E. et al., J. Exp. Bot. 60, 1261-1271 (2009).
24. Hernandez-Onate M. A. et al., Curr Genet 61, 359-372 (2015).
25. Glazebrook J. et al., Annu Rev Phytopathol 43, 205-227 (2005).
26. Mur L. A. et al., Plant Physiol. 140, 249-262 (2006).
27. Derksen H. et al., Plant Sci 207, 79-87 (2013).
28. Dorey S. et al., Plant Physiol. 121, 163-172 (1999).
29. Ashtamker C. et al., Plant Physiol. 143, 1817-1826 (2007).
30. Cui H. et al., New Phytol 213, 1802-1817 (2017).
31. Wan L. et al., Science 365, 799-803 (2019).
32. Rasul S. et al,. Plant, Cell & Environment 35, 1483-1499 (2012).
33. Yun B. W. et al., Nature 478, 264-268 (2011).
34. Ferrari S. et al., Front Plant Sci 4, 49 (2013).
35. Sabatini S. et al., Cell 99, 463-472 (1999).
36. Fan M. et al., Plant Cell 26, 828-841 (2014).
37. Morales M. . et al., Trends Plant Sci 21, 996-999 (2016).
38. Neuser J. et al., Cell Rep 28, 1670-1678.e1673 (2019).
39. Besson-Bard A. et al., J Exp Bot 59, 3407-3414 (2008).