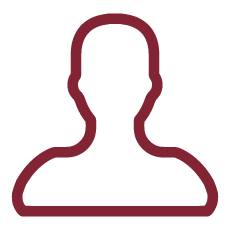
This project will focus on the geochemical evolution of olivine from primitive skarn environments. Magma-carbonate interaction will be investigated by a vertical tube CO¿CO2 gas-mixing furnace. Atmospheric pressure experiments will be carried out at 1250, 1200, and 1150 °C under QFM buffer. The starting materials will be three synthetic basalts (i.e., meltMg#78, meltMg#75, and meltMg#72) doped with variable amounts of CaCO3, in order to reproduce the natural concentration levels of CaO-rich magmas interacting with the skarn rock shells. Results from decarbonation experiments will evidence as the crystallization of MgO-CaO-rich, NiO-poor olivines can be potentially more favored at high temperature, when primitive basaltic magmas assimilate increasing amounts of carbonate materials. This will provide important constrains on the partitioning behavior of major and trace elements and on the formation of ore deposits hosted by skarn environments. In fact, the number of large size Ca cations entering olivine crystal lattice is known to be proportional to the amount of Ca-O-Si bonds available in the melt. Due to differences between Fe2+ and Mg cation radii, the Ca-Fe2+ substitutions into M2 crystallographic site will be more facilitated than Ca-Mg ones, thus enhancing the MgO in olivine. The partitioning behavior of trace elements between olivine and decarbonated melts will be investigated to quantify cation redistribution mechanisms at the magma-carbonate reaction zone. Under the effect of CaCO3 assimilation, the partitioning of divalent and trivalent cations will be parameterized as a function of temperature, bulk composition (CaO and MgO) and melt structure (NBO/T). I will also investigate as cation exchange reactions can be controlled by the strong depolymerizing effect of CaCO3 assimilation that, in turn, is expected to increase the number of structural sites critically important to accumulate rare and precious cations in the melt phase.
Several petrological studies have documented in detail that magma-carbonate interaction is not a rare process (Wenzel et al. 2002; Barnes et al. 2005; Gaeta et al., 2009; Deegan et al., 2010; Mollo et al., 2011). Commonly, magma-carbonate interaction produces an increase of the alkali concentration in melts and provides the development of a skarn rock shell at the periphery of the magma chamber. If carbonate contamination proceeds for a long period, the formation of endoskarn rocks at high temperatures and low oxygen fugacity occurs. These conditions favor the massive crystallization of large olivine crystals and the consequent CaO-enrichment of the residual glass, responding to the complex interplay among metasomatic, metamorphic, and magmatic reactions (Barnes et al., 2005; Chadwick et al., 2007; Gaeta et al., 2009; Di Rocco et al., 2012).
More specifically, olivine crystallization in endoskarn and cumulate environments plays a key role to better understand and quantify the partitioning behavior of major and trace elements. In fact, the simple structure of olivine crystals is composed by a tetrahedral site (T) and two octahedral sites (M1 and M2), that host divalent cations. However, the small dimension of the less distorted octahedra (e.g., Papike et al., 2005) does not facilitate the incorporation of trace elements with large ionic radii or different valence states. As an example, the ionic radius of the Rare Earth Elements (REE) ranges from 1.032 to 0.861 Å, from the Lanthanum (La) to the Lutetium (Lu), respectively. Furthermore, the oxygen fugacity widely changes from the high temperature to the low temperature domains, controlling the valence state of several major and trace elements (e.g., Fe, Mn, V, Cr, Ni) with strong variation of the partitioning of the element between olivine crystal lattice and decarbonated/desilicated melts. As a consequence, important concentrations of trace elements may be accumulated in the CaO-rich SiO2-poor residual melts.
Therefore, a selection of well-established and cutting-edge methodologies in the research field of geochemistry, mineralogy and petrology would be crucial to provide the background data for exploitation of ore deposits. Despite the fact that precious and rare metals and semi-metals (e.g., Au, Ta, Nb, Te, Se, Ge, In, Ga and REE) are accumulated in magma-carbonate environments, the origin of ore-type rocks has been a matter of debate for long time (Geijer, 1917; Parak, 1975; Hitzman et al., 1992; Nyström and Henriquez, 1994; Barton and Johnson, 1996; Sillitoe and Burrows, 2002). Several models have been suggested to explain the genesis of these deposits invoking volcanism, deep plutonism, regional metamorphism, magma-crust interaction, and hydrothermal alteration. In addition, several controversies exist on the main petrogenetic mechanisms that may produce skarns and their associated ore deposits.
In this scenario my project will provide, for the first time, fundamental thermodynamic models to predict the physico-chemical conditions controlling the extraction and accumulation of economically-important amount of rare elements. Furthermore, this study may represent a milestone to quantify the geochemical behavior of rare and precious trace elements during the first stages of the assimilation of carbonate materials by magma.
Barton, M.D., Johnson, D.A., (1996), Evaporitic source model for igneous-related Fe oxide (REE-Cu-Au-U) mineralization. Geology, 24, 259-262
Chadwick, J.P., Troll, V.R., Ginibre, C., Morgan, D., Gertisser, R., Waight, T.E., Davidson, J.P., 2007. Carbonate assimilation at Merapi Volcano, Java, Indonesia: Insights from crystal isotope stratigraphy. J. Petrol. 48, 1793¿1812
Deegan, F.M., Troll, V.R., Freda, C., Misiti, V., Chadwick, J.P., McLeod, C.L., Davidson, J.P., (2010), Magma¿Carbonate Interaction Processes and Associated CO2 Release at Merapi Volcano, Indonesia: Insights from Experimental Petrology. Journal of Petrology, 51, 1027-1051
Geijer, P., (1917), Falutraktens berggrund och malmfyndigheter: Swedish Geological Survey, series C, 275, pp. 319
Hitzman, M.W., Oreskes, N., Einaudi, M.T., (1992), Geologic characteristics and tectonic setting of Proterozoic iron oxide (Cu-U-Au-REE) deposits. Precambrian Research, 58, 241-287
Nyström, J.O., Henriquez, F., (1994), Magmatic features of iron ores of the Kiruna type in Chile and Sweden: Ore textures and magnetite geochemistry. Economic Geology, 89, 820-839
Papike, J.J., Karner, J.M., Shearer, C.K., 2005. Comparative planetary mineralogy: Valence state partitioning of Cr, Fe, Ti, and V among crystallographic sites in olivine, pyroxene, and spinel from planetary basalts. Am. Mineral. 90, 277¿290
Parak, T., (1975), Kiruna Iron Ores Are Not "Intrusive-Magmatic Ores of the Kiruna Type". Economic Geology, 70, 1242-1258
Sillitoe, R.H., Burrows, D.R., (2002), New field evidence bearing on the origin of the El Laco magnetite deposit, northern Chile. Economic Geology, 97, 1101-1109