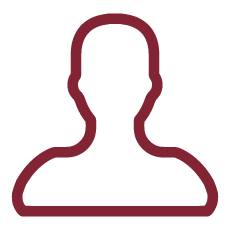
The proposal concerns development and modelling of a microfluidic platform to study cavitation enhanced endothelial permeability within the larger context of targeted drug delivery. The primary barrier drugs need to overcome to reach the target from the circulation is the endothelium which prevents drug from diffusing to the tissues. Cavitation enhanced permeability consists in injecting stabilised microbubbles which are locally activated by ultrasound. The ensuing dynamics exerts mechanical stress on the endothelium and facilitates the opening of the junctional complexes that held tight together the endothelial cells. The interest is motivated by the reversibility of the alteration, with permeability increased for a finite time window followed by recovery of preexistent physiological conditions. Improper protocols may however lead to endothelial damage and cell apoptosis. Hence the interest in a system where mechanical and biological parameters can be held under strict control. The platform we will realise consists of microfluidic channels functionalized with an endothelial layer grown under physiological shear rate and integrates microbubble generators able to finely control dispersity and properties of the injected microbubbles. In parallel, a novel photonic sensor is developed to measure the cavitation induced stress. The project is completed by the theoretical/numerical study of the essential aspects of cavitation enhanced permeability. The phase-field approach is exploited to model cavitation accounting for bubble coatings and the bending rigidity of the endothelium. The proposal exploits the PI's unique expertise in the field, testified by prestigious international awards like the ERC Adg Grant 2013 and the ERC Proof of Concept 2017 INVICTUS concerning cavitation in general and, more specifically, in bio-inspired microchips.
Disorders of the central nervous system cost annual 800 billion euros to the European Health System. Still, advances in drug development are limited since compounds with demonstrated efficacy in animal models are often ineffective in humans. Many failures are due to inability in crossing biological barriers. It is desirable to have cost-efficient tools to modify and measure barrier permeability before the expensive and time-consuming in vivo studies. In vitro experiments can be used to prioritise investigational compounds for in vivo studies or to exclude very poor permeable compounds. They comply with the 3Rs recommendations:``Replace the use of animals in research; Reduce the number of used animals; Refinement, alleviate/minimise pain and enhance animal welfare.
The present proposal builds on previous PI's projects, e.g. the competitive ERC-AdG 2013 'BIC - Cavitation across scales: Following Bubbles from Inception to Collapse' (2.5 M€ over a five year range) centred on fundamental models for cavitation. The techniques developed to understand the effect of cavitation on surfaces and membranes can be exploited for cavitation-enhanced drug delivery, Kooiman et al., Advanced Drug Delivery Reviews, 2014. The ERC Proof of Concept 2016, 'INVICTUS: In vitro cavitation though ultrasound', provided the conceptual layout of the microfluidic platform and its biological functionalisation with a living endothelium that was realised in prototypal form with commercially available elements (e.g. chips by SynVivo and SonoVue echography contrast agents).
The present version of the microfluidic device can advance the understanding of the interaction between cavitating bubbles and biological barriers, primarily the endothelium but also the plasma membrane at single cell level. This powerful tool - to our knowledge, no similar system has ever been realised - can be made turn-key available to researchers in drug delivery. It will open unexpected routes to exploit cavitation enhanced drug delivery. It offers the unique possibility to study the interaction of several kinds of drugs and carriers with different target tissues (diseased and healthy). Experimental simulations of drug delivery can be designed to understand how drugs and drug carriers interact with the endothelial barrier in order to optimise cavitation-based drug delivery protocols. Both extravasation through the endothelial barrier and cellular uptake can be studied in controlled and reproducible conditions, finely tuning the biological, acoustic and fluid mechanical parameters. The system features a realistic shear rate induced by the flow in the blood vessel phantoms, a crucial aspect for the response of the endothelium. The new layout is based on an in-house built microfluidic chip that can be modified according to need and is integrated with the ultrasound emission/reception system. A crucial innovation is the integration of on-board micro-bubble generators to generate microbubble controlled in size and composition, reducing the experimental noise due to the polidispersity of commercial bubbles.
The proposed photonic sensor can measure the stress induced on the wall by cavitating bubbles. The technology, based on Bloch surface waves, is innovative and has never been applied to measure the effect of cavitating bubbles. It can quantify the effect of parameters like bubble dimension, distance from the wall - known from our numerical simulation to be of primary importance for the stress at wall level - and rheological properties of the bubbles. The sensor will be characterised and validated employing Laser induced bubbles that we can control with extreme accuracy in terms of energy and positions. A further step involves its application to precisely positioned microbubble under acoustic irradiation (e.g. via acoustic (Bjerknes) forces or acoustic tweezers).
An atout is the advanced theoretical/numerical modelling of microbubbles encompassing a realistic description of the coat rheology. Experimental results are often difficult to interpret in absence of a good theoretical framework. We expect to develop and use new models of the microbubbles to take into account the bending rigidity of the coating, following the pioneering idea of Helfrich. Our purpose is to embed the rheology of the coatings in a phase field model in order to be able to realistically predict the bubble dynamics under ultrasound irradiation. This advancement will allow to quantify the steady streaming induced by the scattered acoustic field and the shear stress exerted on the endothelium. We also aim at the ambitious goal of capturing the topological changes (from spherical to toroidal) of the coated-bubble expected at high irradiation intensity. The model can be extended to deal with the response of cell membranes to the mechanical stress induced by cavitation following ongoing work carried on in these days by the community.