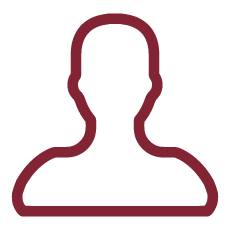
The monitoring of structures with nondestructive techniques is a topical issue in the field of civil constructions. Over the years, modelling and identification procedures have been more and more integrated with each other, so that in standard cases, bridges for instance, structural monitoring activities based on the observation of natural frequencies are part of regular maintenance protocols. The information provided by these techniques derives from the observation of global quantities, so that more detailed information would often be desirable.
In this research project, we address some points that would benefit from further research. We focus on predictive models of structural elements, encompassing effects of prestress, defects, and temperature changes. Our investigations will concern:
-reconstruction of the image of damage, which may be obtained applying ultrasonic imaging techniques similar to those used in medical diagnosis;
-monitoring of the state of stress of structural elements in view of their protection against breakage or buckling, also with regard to states of stress induced by temperature changes.
Advancements in these fields require the development of models able to appropriately describe the structural response, accounting for geometrical and material nonlinearities. This makes possible to describe and investigate non trivial physical phenomena that could point to the best response quantities to be observed in the monitoring activities. These issues will be addressed in this research project within a unified framework encompassing modelling and identification.
The two researchers gathering around this project have fruitfully addressed these subjects in the past years, also with international collaborations. The proponent has consolidated experience in structural modelling and identification. The second member of the research team has a sounding experience in modelling of stability in structural members, either in their damaged or undamaged state.
We focus here on the development of an approach to nondestructive modeling that exploits both local and global response quantities. The areas addressed by the two WPs would benefit from further research and are quite open to advancements of the state of the art.
WP1: Typical imaging techniques use an array of ultrasonic sensors that transmit pressure waves in the inspected medium, and subsequently record the reflections coming from scatterers located in the medium itself (Figure 2). The image can be constructed by extracting selected features from the recorded signals, and by computing the pixel intensities through a beamformer algorithm. All the techniques available in the literature use weighting functions to improve contrast and resolution of the image obtained, but none of them accounts for the actual physics of waves. The proponent has already shown (ref. [2] of section 1) how the introduction of weights based on the wave propagation characteristics can dramatically improve the image of the defect in a plate (Figure 3). What is more, depending on the nature of the excitation force and on the reflector morphology, different wave modes can be excited and reflected, therefore multiple mode combinations can be used in the imaging algorithm. Scatterers like corrosion defects that are typically nonsymmetric are capable to generate a very rich patter of reflections that can be employed to improve the image quality. The compounding of these guided wave mode combinations allows for a further increase of the array gain. A completely new case to be explored concerns orthotropic or anisotropic plate waveguide. This case is actually very common, but not directly treatable with the algorithms valid for isotropic solids. In fact, the wave propagation speed depends on the direction of propagation, and the wavefront is not circular.
Another area of structural damage identification that would profit from further investigations applies image reconstruction algorithms based on tomography. The applications of tomographic imaging within the structural ambit are extremely limited, although they might enable one to literally 3D look into the solids. This could not only be applied to the detection of defects, but also to more ambitious nondestructive investigations providing, for instance, a 3D rendering of steel bars in concrete members or images of pipes embedded in walls or floors. The means to obtain such result would be through the inverse Radon transform, which is used in tissue imaging to reconstruct the unknown density of a tissue. In tissue imaging, the inverse Radon transform is applied to x-rays attenuations, which are not practicable to structures. We claim that similar algorithms could be applied to the propagation of ultrasounds to solids, also possibly exploiting nonlinear response phenomena that may occur in prestressed elements.
WP2: The analytical models used for predictions of changes of the waveguide response following a state of stress evolved from a continuum mechanics model specialized to waveguides. It is a model which accounts for different aspects, that are: non infinitesimal deformations, and the fact the equilibrium takes place in the deformed configuration. A third or fourth order expression of the strain energy of the hyperelastic body is adopted to account for material nonlinearities. Though effective in describing the experimental response, the contribution of each of all those different ingredients has yet to be clarified theoretically. This theoretical standpoint provides the modelling grounds for further investigations on stability. Instability occurs when a parameter of control of the collapse phenomenon (usually a load multiplier) reaches threshold values, called critical, and bifurcation (static or dynamic) phenomena of the response appear; this implies qualitative variations of the response and may cause loss of functionality and reliability of the structural element under investigation. In a more general meaning, starting from a state of equilibrium, a localized or diffused perturbation of some physical properties of a structural element will affect its static and dynamic response. When structural stability depends on more than one parameter, stability maps are needed to assess safety against buckling or flutter (Figure 4, ref. [4] of section 1).
Appropriate measures of this variation have technical interest: with the same approach used to study stability, we mean to evaluate the variation of the response and then grasp how this reflects onto structural safety. Within this framework, we aim to improve current knowledge providing theoretical models richer than those available in the literature, in which damage, temperature variations, initial imperfections and prestress are all taken into account; the results are merged with the outcomes of the local approaches described into WP1 and used to implement novel monitoring and surveillance protocols.