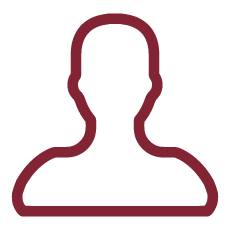
Computer simulations of fluids at the microscale have been thoroughly used in the field of microfluidics for interpretation of experimental observations and for the design of new devices. One of the most used simulation technique is Molecular Dynamics (MD), as it models explicitly every atom of the system, allowing the simulation at nanoscale of complex systems such as biological channels or nanofluidic devices for energy harvesting. The drawback of MD is its computational cost, which makes unfeasible to use it to simulate complete microfluidic devices. At larger scales, continuum computational fluid dynamics techniques are commonly employed. In this case, the main drawback is associated to the lack of thermal fluctuations, which are crucial for correct modelling of several transport phenomena. In fact, most microfluidic systems are mesoscale systems, meaning that some features of the microscopic world as the discrete nature of matter and density fluctuations may be neglected, while other features as thermal motion and the finiteness of the Debye layer can't be neglected. To simulate these systems, coarse grained techniques which drop many unnecessary degrees of freedom, retaining only the relevant features of the system are needed.
The aim of this project is to develop and validate a coarse grained approach able to model the thermal fluctuations, the presence of solid particles immersed in the solution (fluid-structure interactions), the presence of coupled electric and fluid dynamics phenomena (i.e. electrohydrodynamics). The model will be developed in the framework of Dissipative Particle Dynamics (DPD), a mesoscale technique used to model microscale flow. Our main contribution will be to expand it to include ionic fluxes and electric polarization. This expansion will allow to use DPD for a broader class of technologically relevant problems, such as nanopore sensing or energy harversting from a salt gradient.
The possibility of performing DPD simulations of mesoscale systems retaining in a rigorous way important features as electrohydrodynamics or transport of chemical species, would be useful for many of problems in microfluidics and nanofluidics.
As an example, several problems related to nanopore sensing could be addressed by DPD mesoscale simulations. Nanopore sensing is a technique which is used to charachterize biological molecules or other types of nanoparticles immersed in an electrolytic solution [15]. Two chambers separated by a thin membrane with a nanometer sized pore are filled with the electrolytic solution containing the molecules to analyze (the analytes). A voltage difference is applied between the chambers, and the resulting current is monitored. When the analyte translocates from one chamber to the other through the pore, a change in the current is observed, the intensity and the duration of the change depending on the shape, size and charge of the analyte.
At the present, many experiments of very different nanopore systems exist, tackling problems such as DNA and protein sequencing, protein charachterization, study of protein aggregation [16]. The analytical models used to interpret the data coming out from nanopore experiments are often too simple to account for the variety of the possible phenomena occurring (e.g. pore clogging, electroosmotic flow, reduced mobility inside the pore, surface charge effects).
In many cases, a MD approach can be used to study the translocation dynamics and the associated current signal considering the above cited physical effects. These simulations are useful not only to interpret the experimental data, but also to understand the details of translocation dynamics [5], and to investigate the potential effects of a new experimental set up [17]. As these simulations typically employ periodic boundary conditions, the typical dimensions of the systems to be simulated must be much larger than the nanopore size, to avoid the effect of interactions between periodic images of the system during the translocation. This fact restricts the pore dimensions which can be simulated with MD to few nanometers, while larger nanopores are being used for nanopore sensing in the last years [18].
Using CG techniques as DPD would allow a lower computational cost in the simulation of many nanopore systems in which the conformational changes of biological molecules don't have a key role. Moreover, it would allow the possibility to simulate a much larger class of nanopore systems, providing a valuable tool for the design of new nanopore devices and for the interpretation of experimental data. Specifically, the possibility of simulating the exact electrohydrodynamics is of great relevance for nanopore systems, beacuse the presence of charged surfaces has been proved to give rise to peculiar phenomena, such as electroosmotic flow [19], current rectification [20], electrophoretic mobility inversion [21]. At the same time, the dynamics of translocation is influenced by the high geometrical confinement of the analyte in the nanopore.
Concluding, the development and validation of a numerical instrument capable of modelling thermal fluctuations, fluid-structure interactions and electrohydrodynamics would be an important advancement in the field of CG numerical simulations. Moreover, such numerical instrument would allow a quantitative approach for many nanopore sensing problems for which, at the present, a qualitative approach or strong approximations are used. In addition, the usefulness of mesoscale simulations is high in many other fields of microfluidics and nanofluidics in which thermal fluctuations and solid particles are involved and different physical phenomena have to be considered in an unified framework (e.g. electrohydrodynamics [22] or transport and reaction of chemical species [23]).
[15] Shi et al., "Nanopore sensing", Anal. Chem., 2017
[16] Balme et al., "Influence of adsorption on proteins and amyloid detection by silicon nitride nanopore", Langmuir, 2016
[17] Belkin and Aksimentiev, "Molecular dynamics simulation of DNA capture and transport in heated nanopores.", ACS Appl. Mater. Interfaces, 2016
[18] Waduge et al., "Nanopore-based measurements of protein size, fluctuations, and conformational changes.", ACS Nano, 2017
[19] Asandei et al.,"Electroosmotic trap against the electrophoretic force near a protein nanopore reveals peptide dynamics during capture and translocation.", ACS Appl. Mater. interfaces, 2016
[20] Yang et al., "Charge Induced Rectification in Single Nanopores.", Biophys. J., 2016
[21] Luan and Aksimentiev, "Electric and electrophoretic inversion of the DNA charge in multivalent electrolytes." Soft Matter, 2010
[22] Modestino et al., "The potential for microfluidics in electrochemical energy systems.", Energy Environ. Sci., 2016
[23] Epstein and Xu, "Reaction¿diffusion processes at the nano-and microscales." Nat. Nanotechnol., 2016