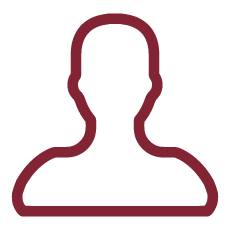
Pseudomonas (P.) aeruginosa antibiotic resistance represents an increasing threat to global public health as prevents the effective treatment of chronic infections of this pathogen, listed in the top six most threatening bacteria by WHO. Therefore, understanding how this bacterial pathogen resists antimicrobials and identifying novel drug targets is crucial for successful therapeutic interventions.
P.aeruginosa deploys several strategies to resist antibiotics, including the endogenous production of hydrogen sulfide (H2S), recently recognized as a general protective gaseous molecule, which renders multiple bacterial species highly resistant to oxidative stress and host immune responses. However, as the other gaseous molecules NO and CO produced by the host to combat infections, H2S interferes with cellular respiration. P.aeruginosa respiratory chain is highly branched and relies on five terminal oxidases: an aa3-, two cbb3-, a bo3-type and a Cyanide Insensitive Oxidase (CIO).
Relevant to human pathophysiology, CIO belongs to the family of bd type oxidases, key respiratory enzymes that confer bacterial resistance to several types of stresses and promote virulence in some pathogens. Our working hypothesis is that the respiratory chain complexes expression is finely tuned during infection and CIO production endows bacterial aerobic respiration with the ability to resist H2S and the other toxic gaseous molecules.
The present project aims at studying the P.aeruginosa terminal respiratory chain complexes to understand the role played by these enzymes within the framework of host-pathogen relationships.
Research will focus on the oxidase expression profile in H2S, NO, CO P.aeruginosa stressed cells and on the effect of these gases on energy metabolism, evaluated in cell membranes and compared to E.coli as a model system, as well as on the isolation and characterization of CIO to shed light on reaction mechanisms and their pathophysiological relevance.
The increasing frequency of antimicrobial resistance in P. aeruginosa represents a great threat to humans, and the absence of definitive pharmacological agents and therapy requires research and characterization of new targets for the development of the next generation of therapeutics (1). Potentiating existing antibiotics by attacking a general defence mechanism of pathogenic bacteria (8) is an attractive strategy to address this need. In this project, we aim at investigating the role of P. aeruginosa respiratory oxidases in bacterial tolerance to H2S, as well as NO and CO, gaseous molecules involved in bacterial protection from antibiotics and host assault [20,23].
While much is known about the role of these gasotransmitters in mammalian pathophysiology, their impact on bacterial physiology has been poorly investigated so far and our understanding of how they exert their regulatory signalling action is elusive as yet, notwithstanding their emerging role in the success of microbial infection.
Intriguingly, they are also potent inhibitors of heme-copper respiratory oxidases and thus modulators of energy metabolism, now recognised to be critically important to the growth and colonization potential of pathogens, even in persisters, metabolically less active and antibiotic resistant population [27].
Presently there is strong interest in exploiting the link between oxidative phosphorylation and pathogenesis, since there is evidence suggesting that energy metabolism might be an important signal used by bacterial pathogens to identify specific host environments. The proportion of the antibiotic-tolerant cells greatly increases after the transition to the stationary phase of growth, where O2 levels and the metabolic rate progressively decreases.
Relevant to human pathophysiology, in P. aeruginosa CIO activity is induced about fivefold upon entry from exponential into stationary phase, when the proportion of persisters increases as well as the amount of H2S [8]. However, no correlation was observed between CIO induction and the low levels of O2 dissolved in the growth medium.
The hypothesis that H2S concentration may act an inducing signal for the expression of a tolerant respiratory oxidase in P. aeruginosa has never been addressed. The biochemical assays proposed in this project will allow us to explore the impact of this gas, as well as NO and CO, in the regulation of the electron transfer chain complexes expression and in cell bioenergetics.
The understanding of how bacterial-generated gasotransmitters regulate energy metabolism as well as the effect of the on bacterial survival, is relevant within the framework of host-pathogen relationships, as it contributes to a broader comprehension of the molecular mechanisms allowing P. aeruginosa host colonization and infection, and it may help to identify key therapeutic targets. Preliminary data indicate a correlation between CIO-dependent respiratory activity and sulphide tolerance.
Since P.aeruginosa CIO has not been isolated yet, our goal is to develop well-designed protocols to express and purify this enzyme to obtain an active form of the protein for the proposed functional and biochemical characterization. Overall, this information will hopefully provide input to new research and future crystallographic studies useful for drug design, with the final goal of discovering oxidase inhibitors able to potentiate the efficacy of companion clinical drugs used against P.aeruginosa while protecting against the emergence of antibiotic resistance.