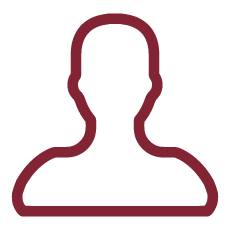
The establishment of a specific nucleoprotein structure, the telomere, is required to ensure the protection of chromosome ends from being recognized as DNA damage sites. In humans, telomeres consist of thousands of TTAGGG repeats bound by a six-protein complex named shelterin. The complete replication of telomeric repeats is assured by the enzyme telomerase, which is active only in germinal and stem cells. In somatic cells, telomeres shorten with each replication round till they reach a critical length leading to a cell cycle arrest known as replicative senescence. Mutations in the growth arrest pathway could allow cells to bypass senescence and continue dividing. Most telomeres uncap, resulting in a crisis stage characterized by chromosome end-fusions and massive apoptosis. To acquire unconstrained proliferative capacity, cancer cells must escape senescence and/or crisis by reactivating telomerase or by alternative mechanisms of telomere maintenance.
Telomeric chromatin has peculiar features: tight nucleosomal structure, enrichment in the histone variant H3.3 and specific heterochromatic histone marks. Mutations in genes responsible for the telomeric epigenetic pattern (SIRT6) or for H3.3 deposition at telomeres (ATRX, DAXX) are deleterious for the stability of human telomeres. Importantly, H3.3/ATRX/DAXX mutations are often found in ALT tumors, suggesting that they play a key role in ALT development.
Despite the essential role telomeres play in cancer development and in aging, our knowledge of the chromatin structure of protected and deprotected telomeres is still insufficient.
In this research project we will address two main issues:
1. The structural and epigenetic changes of human telomeres switching from a protected to a deprotected state.
2. The role played by nucleosomes in telomere capping and how they affect shelterin binding.
REFERENCES
1. Palm, W. and T. de Lange, How shelterin protects mammalian telomeres. Annu Rev Genet, 2008. 42: p. 301-34.
2. Sfeir, A. and T. de Lange, Removal of shelterin reveals the telomere end-protection problem. Science, 2012. 336(6081): p. 593-7.
3. Shay, J.W. and W.E. Wright, Senescence and immortalization: role of telomeres and telomerase. Carcinogenesis, 2005. 26(5): p. 867-74.
4. Maciejowski, J. and T. de Lange, Telomeres in cancer: tumour suppression and genome instability. Nat Rev Mol Cell Biol, 2017. 18(3): p. 175-186.
5. Michishita, E., et al., SIRT6 is a histone H3 lysine 9 deacetylase that modulates telomeric chromatin. Nature, 2008. 452(7186): p. 492-6.
6. Heaphy, C.M., et al., Altered telomeres in tumors with ATRX and DAXX mutations. Science, 2011. 333(6041): p. 425.
7. Mohammad, F. and K. Helin, Oncohistones: drivers of pediatric cancers. Genes Dev, 2017. 31(23-24): p. 2313-2324.
8. Cusanelli, E. and P. Chartrand, Telomeric repeat-containing RNA TERRA: a noncoding RNA connecting telomere biology to genome integrity. Front Genet, 2015. 6: p. 143.
9. Galati, A., et al., TRF1 and TRF2 binding to telomeres is modulated by nucleosomal organization. Nucleic Acids Res, 2015. 43(12): p. 5824-37.
10. Cubiles, M.D., et al., Epigenetic features of human telomeres. Nucleic Acids Res, 2018.
11. Schoeftner, S. and M.A. Blasco, Chromatin regulation and non-coding RNAs at mammalian telomeres. Semin Cell Dev Biol, 2010. 21(2): p. 186-93.
12. Kraushaar, D.C., et al., Genome-wide incorporation dynamics reveal distinct categories of turnover for the histone variant H3.3. Genome Biol, 2013. 14(10): p. R121.
13. Udugama, M., et al., Histone variant H3.3 provides the heterochromatic H3 lysine 9 tri-methylation mark at telomeres. Nucleic Acids Res, 2015. 43(21): p. 10227-37.
14. Schwartzentruber, J., et al., Driver mutations in histone H3.3 and chromatin remodelling genes in paediatric glioblastoma. Nature, 2012. 482(7384): p. 226-31.
15. Lovejoy, C.A., et al., Loss of ATRX, Genome Instability, and an Altered DNA Damage Response Are Hallmarks of the Alternative Lengthening of Telomeres Pathway. PLoS Genet, 2012. 8(7): p. e1002772.
16. Chu, H.P., et al., TERRA RNA Antagonizes ATRX and Protects Telomeres. Cell, 2017. 170(1): p. 86-101 e16.
17. Kim, H., et al., Systematic analysis of human telomeric dysfunction using inducible telosome/shelterin CRISPR/Cas9 knockout cells. Cell Discov, 2017. 3: p. 17034.
18. Arnoult, N., A. Van Beneden, and A. Decottignies, Telomere length regulates TERRA levels through increased trimethylation of telomeric H3K9 and HP1alpha. Nat Struct Mol Biol, 2012.
19. Koering, C.E., et al., Human telomeric position effect is determined by chromosomal context and telomeric chromatin integrity. EMBO Rep, 2002. 3(11): p. 1055-61.
20. Lowary, P.T. and J. Widom, New DNA sequence rules for high affinity binding to histone octamer and sequence-directed nucleosome positioning. J Mol Biol, 1998. 276(1): p. 19-42.
21. Carey, M.F., C.L. Peterson, and S.T. Smale, In vivo DNase I, MNase, and restriction enzyme footprinting via ligation-mediated polymerase chain reaction (LM-PCR). Cold Spring Harb Protoc, 2009. 2009(9): p. pdb prot5277.