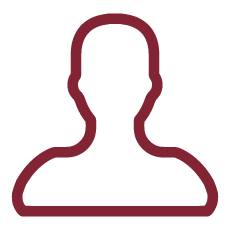
A glass is a microscopically disordered solid-like state which can be achieved by any substance, provided that crystallization is avoided by cooling or compression. Simple and molecular liquids, polymers, bio-materials, metals and molten salts can form glasses, thus making the glass transition a rather ubiquitous phenomenon in condensed matter physics. From the phenomenological point of view, on approaching the glass transition the relaxation time of the system increases by many orders of magnitude and the material starts behaving like a solid, although microscopic translation and orientational order is absent. At present, a full theoretical understanding of the glass transition is still lacking.
A recent and very promising route to produce glassy states is provided by chemical vapor deposition, a technique through which it is possible to generate glasses that are extremely stable both thermodynamically and kinetically. In this project we will use molecular dymamics simulations to apply the chemical vapour deposition protocol to water, which is one of the most studied glass-forming substances. We plan to compare the thermodynamic and kinetic properties of the resulting material with the behaviour of the two different forms of glasses that water produces, i.e. the low-density amorphous and the high-density amorphous ices, whose main properties are connected to the anomalous thermodynamic behaviour of water. We will do so by employing recently developed numerical methods based on high-dimensional order parameters, combined with artificial neural networks classification schemes. The final goal of the project is to assess the stability of the glassy water obtained with different water and water-like models, and to characterize its thermodynamic and kinetic properties.
The proposed research aims at significantly advancing the state-of-the-art in the preparation and characterization of chemically deposited ices. Current efforts in the simulation of chemical vapor deposition have been limited to simple spherical symmetric potentials. In our study, we will systematically explore the mechanism of ultra-stable glass formation in systems with hydrogen bonds. Advanced numerical methods will be employed to numerically simulate the deposition of amorphous ices on different substrates. The water potential will be altered with Hamiltonian integration techniques to assess the role of tetrahedrality on the formation of amorphous layers. The results will then be compared to bulk ices and will allow us for the first time to structurally compare ices formed with different protocols.
To analyse the amorphous structures, we will design and employ new classification algorithms based on neural network approaches, that so far have not been applied to vapor deposition. In particular we will introduce new structural descriptors for the characterization of local structures in tetrahedral networks. These descriptors will be optimized for distinguishing HDA and LDA amorphous phases, and will take advantage of our unique experience in the numerical simulation of bulk amorphous phases of water. The resolution and accuracy of the new classification methods made possible by deep neural networks will allow us to characterize these glasses also in the presence of density perturbations induced by the free surface, which was not possible before.
We expect our results to shed new light on the mechanism of formation of amorphous ices, and on the role played by the free surface in the relaxation towards ultra-stable states. We will be in the unique position to test the hypothesis that vapor deposited amorphous glasses are connected to the LDA phase, and help understand the results of experiments whose interpretation has remained controversial for the lack of comparisons with theoretical models.
Our results will be relevant to both scientific communities studying glasses and water. Varying tetrahedrality in our models will also allow us to understand the mechanism of ultra-stable glass formation in different tetrahedral materials with high technological impact, such as silicon and germanium.