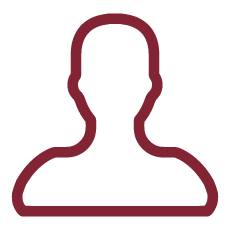
In two-dimensional (2D) crystals, mechanical deformations turn out to be a powerful tool to control the material's properties thanks to the strong interplay between the lattice and the electronic band structure.
Here, we focus on a novel route to strain-tune the electronic properties of transition metal dichalcogenides (TMDs), a class of layered semiconductors with chemical formula MX2 (where M: W or Mo and X: S or Se) that can be exfoliated down to a single X-M-X layer. Our method is based on the capability to deform at the micro- and nano-scale a single X-M-X plane due to the deliberate trapping of molecular hydrogen in between adjacent layers in bulk flakes. Specifically, by proton irradiation we create atomically-thin TMD domes filled by H2 with internal pressure ~10 atm. The domes feature intense light emission and can be site- and size-controlled from 10000 nm to 50 nm. Here, we aim at a detailed characterization of the electronic properties of single and ensembles of WS2, WSe2, MoS2 and MoSe2 domes by spatially-resolved (500 nm resolution) photoluminescence, Raman scattering, photon-correlation and second-harmonic generation spectroscopies. X-ray photoemission spectroscopy of the M and X elements will monitor the decoupling of the upper TMD layer from the underlying parent substrate and provide information about the structural modifications caused by the lattice deformation ensuing the gas internal pressure. The goal of this project is twofold. First, we will address open issues regarding the electronic structure of strained 2D TMDs and hence explore the attainment of quantum confinement effects for the realization of single-photon sources at room temperature. Second, these studies will be instrumental for advanced investigations by photoemission spectromicroscopy (spatial resolution
Most of the methods addressing the effect of strain on 2D crystals rely on the use of bulky bulging devices to sustain the gas inside the deformed layers [18,33], or need electric [34] or thermal [20] fields to stress controllably the atomic planes. Other approaches exploit the spontaneous formation of gas bubbles between a single layer and the substrate beneath [22]. By our method, instead, no external device is required and the dome can be created in a site-controlled fashion as demonstrated in Fig. 6. In turn, this will allow us to characterize the domes by diverse techniques in different laboratories. On 2019 we have scheduled photoemission spectromicroscopy measurements with spatial resolution below 100 nm on a single dome. This study will be performed at the ANTARES beamline of the Soleil synchrotron. Our goal is the determination of the energy dispersion of the electronic states of WS2 or WSe2 domes, and in particular the evolution of the relative energy at the K and Gamma points of the valence band, a topic largely investigated theoretically [6,13,33] but poorly characterized experimentally despite its enormous impact for photovoltaics and lighting applications. The unique spatial resolution (
Similarly important, micro-magneto-PL up to 30 T (for which we apply at the European Magnetic Field Laboratory on May 2018 and scheduled the experiments on 2019) will permit us to map for the first time the evolution of the exciton properties at different degrees of strain. Indeed, the combination of strain and magnetic field may unveil peculiar interaction/anticrossing mechanisms between valence band states with different symmetry and valley index, which will be identified via polarization-dependent magneto-micro-PL. This study has never been performed before due to the typically small sample space available in high magnetic field cells that require tiny, compact samples or devices. We stress that the need for high magnetic fields is dictated by the typically large effective mass of carriers (~0.2 m0, where m0 is the electron mass in vacuum) and correspondingly very small exciton size (~2 nm) in TMD MLs that usually leads to diamagnetic shifts as small as few meV even at 65 T [24].
The investigation of the effects of strain -and, for the smaller structures, of quantum confinement- on the electronic and optical properties of 2D TMD micro- and nano-domes may represent an important advancement in the field of 2D crystals. Indeed, while this project surely addresses a topic of great fundamental interest -especially as it regards the hitherto unobserved direct-to-indirect transition, which is expected to take place (for ~2% biaxial strain) in strained TMD nano-domes- its main appeal is probably rooted in the possibility of exploiting these effects to finely tune the light-emitting properties of the fabricated nanostructures. This latter goal suits the newly launched Flagship on Quantum Technologies, according to which (https://tinyurl.com/QT-HLSC-report) the development of triggered, site-controlled single-photon sources would be beneficial to many of the overarching goals of the Flagship, such as the development of Quantum Simulators with a certified quantum advantage (wherein ordered arrays of single-photon sources will be required). Within this framework, the perspective goal of the present project, i.e., the development of room-temperature single-photon sources based on size- and position-controlled nano-domes, would be hailed as a major milestone. This is particularly true at the light of the inherent integrability of these nano-domes with silicon-based nanophotonic and nanoplasmonic devices. On the one hand, such integration will allow us to further control and optimize the properties of our light emitters -particularly in terms of their radiative recombination rate (Purcell Effect) and light extraction efficiency [35] - eventually enabling the realization of advanced light-emitting devices, e.g., nanolasers [36] . On the other hand, the possibility to deterministically integrate site-controlled single-photon emitters on advanced photonic structures, compatible with (Si-based) current electronic devices, is one of the key goals of the Quantum Technologies Flagship, particularly as it regards Quantum Computation and Quantum Simulation.