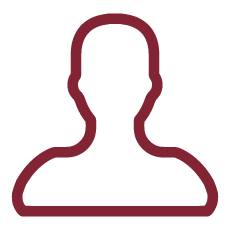
The implementation of a quantum network is a mandatory requirement for the full development of quantum information, computation, and information sciences. The accomplishment of this task requires single photon emitters, which should emit on-demand single photons and, potentially, entangled photon pairs. Semiconductor quantum dots, also dubbed artificial atoms, dots are currently emerging as near-ideal single-photon sources but, after almost 20 years of extensive efforts, bringing them out to the market place remains still a grand challenge, since there are many difficulties linked to the scalability requirement set by quantum technologies. On the other hand, it has been recently demonstrated that localized excitons in 2-dimensional transition metal dichalcogenides can emit single photons with very high brightness. It has been shown that the origin of this new kind of solid-state quantum emitter is linked to the presence of strain fields, that favours exciton funnelling towards low-energy bandgap regions, where single localized excitons can be populated. In turn, this has led to the formation - in a moderately easy way - of site-controlled quantum emitters. Despite the pioneering experiments in this field, a detailed description focused on the specific role played by strain in the quantum emitter formation is still lacking in the literature. In this project, a systematic study of the role of strain, which will be varied dynamically via nano-machined piezoelectric actuators, on the quantum emitter formation will be performed. A single layer of WSe2 will be deposited on piezoelectric nano-pillars, on which strain fields can be modified dynamically, and by means of confocal photoluminescence mapping and atomic force microscope measurements, the role of strain in the existence of single photon sources in 2-dimensional material will be investigated.
When strained TMD MLs are cooled-down to cryogenic temperatures, localized excitons appear in the optical spectrum. Several works [1] have demonstrated that these excitons behave as single photon sources that can be potentially used for quantum communication, and therefore as building blocks of a quantum network. Single emitters in TMDs have several advantages indeed compared to other sources of non-classical light, such as QDs. They can be created using simple and cost-effective methods, their nucleation site can be controlled with nanometric precision, and they do not suffer from hurdles related to total internal reflection. Despite the physical origin of TMD quantum emitters is still under debate [2], some works [3, 4, 5] have demonstrated that their appearance is tightly connected to spatial strain gradients (usually arising from wrinkles or buckles) which (most probably) favor exciton funnelling from high-energy towards low-energy bad gap regions where single localized excitons can be efficiently populated [3].
In this regard, this project will study the wrinkle geometries and follow, by means of low-temperature -PL spectroscopy and AFM measurements, their evolution as the voltages applied to the nano-machined piezoelectric nanopillars are varied. Indeed, albeit it is widely accepted that strain induced by wrinkles or buckles plays a crucial role in the formation of TMD quantum emitters, no group so far had the possibility to change the wrinkle configuration dynamically at cryogenic temperatures. Therefore, we will investigate how strain gradients can lead to the appearance of localized excitons in SL TMDs. Besides, we will use strain to engineer the emission properties of single emitters in TMDs, with particular focus on the possibility to control the energy and the polarization of the emitted photons, as already demonstrated by our group in the QD community [6]. Previous results based on monolithic actuators (i.e., not nano-machined) show that the idea is feasible, and that strain induced by a voltage difference can be successfully delivered to a WSe2 SL [7]. This will allow us to demonstrate for the first-time energy-tunable single-photon source based on 2D-materials. Moreover, thanks to the expertise owned in our group of advanced quantum optics techniques such as photon correlation spectroscopy, quantum state tomography, and Hong-Ou-Mandel two-photon interference, a complete characterization of the quality of the emitted photons will be performed. Once a full characterization of the source¿s figures of merit will be performed, an accurate comparison between this new kind of solid-state single photon emitter and QDs could be made, in order to establish if there are actual relevant advantages with respect to the well-established QD source.
Another key ingredient of the project is given by the fact that quantum networks demand ¿near-ideal¿ sources of photons combined with quantum memories [8]. Semiconductor QDs would embody such an ideal source but interfacing single photons with existing quantum memories [9] turned out to be challenging. Other approaches based on spin-photon interfaces are still limited by the short spin coherence times of electrons and holes. On the other hand, instead, the emission and spin properties of localized excitons in TMDs are mostly unexplored, and the research that will be carried out in the present project might open new routes for quantum technologies. Finally, a natural step beyond will be the demonstration of site-controlled single photon emitters in TMDs integrated on state-of-the-art photonic cavities.
[1] V. Perebeinos, Nat. Nano. 10 485 (2015).
[2] Y.J. Zheng et al., ACS nano in press (2019).
[3] A. Branny et al., Nat. Comm. 8 15053 (2017).
[4] C. Palacios-Berraquero et al., Nat. Comm. 8 15093 (2017).
[5] P. K. Chow et al., ACS Nano 9, 1520 (2017).
[6] Trotta R. et al., Nat. Com. 7, 10375 (2016).
[7] J. Martín-Sánchez et al., Semi. Scie. and Tech. 33 013001 (2017).
[8] S. Wehner et al., Science 362 303 (2018).
[9] J. Wolters et al., Phys. Rev. Lett. 119 060502 (2017).