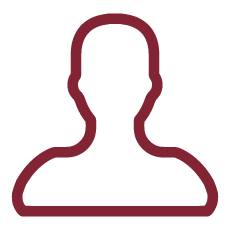
From a Physics and Engineering standpoint, swimming bacteria are a formidable example of self-propelled micro-machines. Together with their synthetic counterpart, self-propelled colloids, they represent the "living" atoms of active matter, an exciting branch of contemporary soft matter and statistical mechanics. Differently from synthetic colloids, however, each bacterial cell contains all the molecular machinery that is required to self-replicate, sense the environment, process information and compute responses. Breaking down these biological functions into basic genetic parts has been one of the greatest triumphs of molecular biology. Today, synthetic biologists are assembling these parts into new genetic programs to exploit bacteria as computing micro-machines.
Project SYGMA will employ the synthetic biology toolkit to provide the building blocks for a light controllable active matter having reliable, reconfigurable and interactively tunable properties. Present active matter is mainly focused on controlling collective behavior by shaping the physical world outside active particles. SYGMA will move instead towards a completely different direction, where a deeper control on shape, structure and dynamics will be achieved by using light to remotely control the biological degrees of freedom inside each cell.
The project is articulated into three main work packages:
1. Light sensors for tunable dynamical response in single cells
We will use engineered photoreceptors to control speed, tumbling, growth and death rates
2. Biohybrid micro-machines with independent degrees of freedom
Teams of micro-shuttles propelled by bacteria will be guided independently by encoding motion directives into the spatial and colour structure of illumination light.
3. Optical control of population shape, structure and dynamics
By projecting a colour movie on a layer of genetically engineered bacteria we will control the shape, structure and dynamics of swimming and colony forming bacteria.
This research project will move first important steps in a direction that will be crucial for the future of active matter physics. Coupling self-propulsion to internal state variables (that are controllable with external fields or through the exchange of messages with other agents) will enable new phenomena and new technologies that will challenge researchers in both fundamental and applied physics for many years.
- Fundamental physics.
Condensed matter physics typically deals with indistinguishable atoms that passively bump on each other exchanging mechanical energy. The inclusion of "activity" has allowed to generalize established physical theories for liquid crystals or spin systems, to successfully describe phenomena that traditionally fell outside the boundary of physics, like active nematics or flocking. Breaking the "indistinguishability" assumption and allowing for internal degrees of freedom will dramatically expand the range of possible behaviors allowing for the formation of complex and programmable patterns and shapes. This will require, however, a big effort in theoretical modelling, which can be successfully undertaken only once we will have the possibility of performing reproducible and controlled experiments like the ones we propose here. The main challenge from a fundamental physics standpoint is that of providing a coarse grained model describing the density dynamics of bacteria with light dependent motility and moving over a structured light pattern. The model should be capable of predicting quantitatively the stationary states of bacteria concentration in static patterns. More interestingly, analysis of time varying responses could lead to the formulation of an "active matter" analog of the optimal protocols for the swift relaxation to equilibrium [1]. This will allow to produce large scale rearrangements of bacteria in short time with applications to micromanipulation, physical and chemical patterning at the micro scale.
- Applied physics
In the modern era of molecular biology, Nature revealed a "treasure" of biological nano-machines whose function and performance have been shaped by evolution since life began on this planet. Through joint efforts, like the one we propose here, putting together physicists, engineers and biologists, we will learn to exploit this "catalogue" of parts to construct versatile, reliable and controllable mechanical components. Only then, hybrid micro-machines will start to have a real impact on lab-on-chip technologies and allow to automate complex tasks like sorting, transport, or cell manipulation for selective diagnostic screening through
single cell genomics and proteomics [2].
[1] Nature Physics 12, 843 (2016)
[2] PLoS genetics 10, e1004126 (2014)