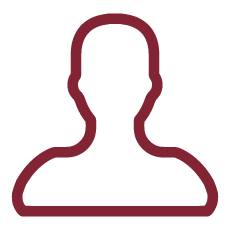
There is a growing interest in functionalizing graphene (Gr), the prototype one-atom-thick two-dimensional (2D) material, in order to obtain a semiconducting 2D material with the excellent mechanical and morphological properties of graphene. A fully hydrogenated graphene mesh is called "graphane" and it is predicted to present an energy gap above 3.5 eV, maintaining the sheet properties of graphene, with a distortion of the sp2 bonds towards sp3 due to C-H bonding, whose realization would have a high impact in opto-electronic devices. Experimental achievement of "graphane" with a high H-upload and a low density of defects is still an open problem, though. Furthermore, it has been recently proposed a new high-resolution detector whose core element is tritium (T) bonded to Gr, but the manipulation of T presents severe safety limitations.
In this project we propose a new path to realize "graphane" by bonding of atomic hydrogen (H) and deuterium (D), in a very compact Gr 3D architecture, nano-porous graphene (NPG), which limits the presence of defects with respect to Gr flakes, transferred Gr etc. NPG enhances the surface-active areas ensuring a topological structure with highly connected layers, negligible density of lattice and edge defects, to engineer physical and chemical properties for the desired functionalities.
We will synthesize "graphane" by low-energy ion and hot inlet tube, at increasing H and D doses, and study it by combining x ray photoemission (XPS), Raman and IR, to determine the electronic and optical properties of 3D graphane. We will also exploit synchrotron radiation (SR) spatially-resolved photoemission at SR facilities, where the participating groups are involved in the research and development activities. We will study the chemical bond, the concentration of H and D upload, and the stability of "graphane", also pointing at enlightening possible isotope dependences, of paramount importance in view of the perspective T application.
Achievement of semiconducting graphene ("graphane") in a purely self-standing 2D continuous sheet, with low defect density, is a very important goal. It can pave the way for future applications integrating the 2D sheet into plastic opto-electronic devices. Since now, the energy gap opening has been obtained only at Gr nanoribbons or size-confined Gr, at the cost of very high defect density. This poses severe limits in the use of "graphene", at contrast with the inter-connected continuous NPG, with a planar shape but pinned sp3 bonding in the honeycomb lattice.
A fine measurement of the sp3 component in the C 1s core level will allow to directly estimate the percentage of sp3-to-sp2 bonds, thus of the at. percentage uptake of H (and D), and to test the thermal stability by progressive heating till disappearance of the sp3 spectral feature.
Till now, the search for experimentally obtain ¿graphane¿ were performed mostly on metal-supported Gr [luo2010, haberer2011, balog2013], which exposes only one face to the decoration of H, thus intrinsically limiting the density of H-C bonds in the layer, or on transferred Gr flakes [burgess2011, whitener2014, son2016] with a high density of lattice and edge defects. With this project we aim at exposing graphene to atomic H and atomic D on both faces, thanks to our experience acquired in the recent years in the use of micro- and nano- porous self-suspended graphene.
With the method proposed in the present project, hydrogenation (deuteration) will cause H (D) bonding to C on both faces of NPG and MPG samples, so to achieve a high density of sp3 bonds while maintaining the average 2D layer structure of the graphene sheet. We will also technologically develop an in-situ method of H (D) uptake, by designing, mounting and testing a complete ultra-high-vacuum (UHV) compatible H source. Eventually, we will determine the thermal stability of actual free-standing "graphane" with a low defect density and high H (D) upload. These achievements constitute an advanced goal in the realization of unprecedented high-quality "graphane" sheet.
[balog2013] R. Balog, M. Andersen, B. Jørgensen, Z. Sljivancanin, B. Hammer, A. Baraldi, R. Larciprete, P. Hofmann, L. Hornekær, S. Lizzit, ACS Nano 7, 3823-3832 (2013)
[luo2010] Luo et al., Appl. Phys. Lett. 97, 233111 (2010)
[haberer2011] Haberer et al., Phys. Stat. Sol. B 248, 2639 (2011)
[sofo2017] Sofo et al., Phys. Rev. B 75, 153401 (2007)