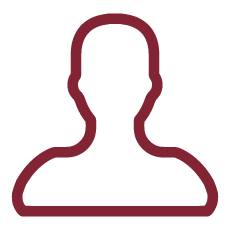
3-Aroyl-1,4-diarylpyrroles are potent inhibitors of tubulin polymerization and cancer cell growth by binding the colchicine site of tubulin, recently developed by our research group. The most potent derivative inhibited the proliferation of BCR/ABL-expressing KU812 and LAMA84 cells from chronic myeloid leukemia (CML) patients in blast crisis and of hematopoietic cells ectopically expressing the imatinib(IM)-sensitive KBM5-WT or IM-resistant KBM5-T315I mutation. The same derivative minimally affected the proliferation of normal blood cells, indicating that it may be a promising hit compound to overcome broad tyrosine kinase inhibitor (TKI) resistance in relapsed/refractory CML patients.
Preliminary results suggested that the introduction of a heterocycle at position 4 of the pyrrole ring increase both tubulin polymerization and antileukemia activity. The present research aims to identify one or more new lead compounds endowed with improved biological and pharmacokinetic profile by introducing on the pyrrole ring different heterocycles at position 4 and substituting the phenyl ring at position 1 with electron donating and withdrawing groups.
All the newly compounds will be tested for their ability to inhibit tubulin polymerization, binding of colchicine to tubulin and cancer cell growth. The most active compounds as single agent or in combination with other TKIs will be then investigated for efficacy with BCR/ABL-expressing cells established in vitro from CML patients in blast crisis (KU812 and LAMA 84), using normal blood cells as control. The analysis will be extended to proliferation and viability of IM-sensitive KBM5-WT and IM-resistant KBM5-T315I cells. The anticancer activity of the same compounds will be evaluated in other TKI-resistant leukemia cell lines. The biological profile of the new derivatives will be further investigated in terms of influence on mitochondrial functions, effects on cell life/death processes and pharmacokinetic profile.
Targeted therapy in the form of selective tyrosine kinase inhibitors (TKIs) has transformed the approach to management of chronic myeloid leukemia (CML) and dramatically improved patient outcome to the extent that imatinib is currently accepted as the first-line agent for nearly all patients presenting with CML, regardless of the phase of the disease [1].
Impressive clinical responses are obtained in the majority of patients in chronic phase; however, not all patients experience an optimal response to imatinib, and furthermore, the clinical response in a number of patients will not be sustained. The process by which the leukemic cells prove resistant to TKIs and the restoration of BCR-ABL1 signal transduction from previous inhibition has initiated the pursuit for the causal mechanisms of resistance and strategies by which to surmount resistance to therapeutic intervention. ABL kinase domain mutations have been extensively implicated in the pathogenesis of TKI resistance; however, it is increasingly evident that the presence of mutations does not explain all cases of resistance and does not account for the failure of TKIs to eliminate minimal residual disease in patients who respond optimally [1].
The limitations of imatinib have inspired the development of second (dasatinib, nilotinib, bosutinib, and bafetinib) and third generation (ponatinib) TKIs in order to overcome the effect of resistance to this primary therapy. These new TKIs have been developed and each has shown efficacy against various common mutant forms of BCR-ABL. This leads to the observation that the various mutant forms of BCR-ABL result in CML that have unique dynamics under therapy, and that combinations of these inhibitors may be necessary to effectively control a rapidly evolving CML population. Patients with CML often die due to transformation of the disease into an acute form of leukemia known as blast crisis. It has been shown that blast crisis is due to the accumulation of additional mutations in CML progenitor cells [2].
The above-mentioned concerns highlight the pressing need for devising new combination regimens aimed at overcoming TKI-resistance in CML cells causing their permanent growth arrest or alternative (e.g., BCR/ABL kinase-independent) non apoptotic cell death mechanisms. Accordingly, because of their crucial roles in the maintenance of cell shape, cell motility intracellular transport, and the formation of the mitotic spindle during cell division, microtubules are emerging as highly attractive target for the development of effective antileukemia agents [3,4]. Although not new, the use of a colchine site binding agent as arm to treat leukemia remains not fully explored.
Taking into account the very interesting results obtained by recently developed 3-aroyl-1,4-diarylpyrroles (ARDAPs) as inhibitor of tubulin polymerization and anti-leukemia agents,[5] the present research aims to identify one or more new lead compounds endowed with improved biological and pharmacokinetic profile by introducing on the pyrrole ring different heterocycles at position 4 and substituting the phenyl ring at position 1 with electron donating and withdrawing groups. We expect to achieve a new drug for the treatment of leukemia with improved cytogenetic and molecular responses in patients with BCR-ABL dependent or independent mechanisms of resistance. The associations of an ARDAP compound with a TK inhibitor have potential to be an improved therapeutic treatment for patients in accelerated/blast phase. ARDAP derivative could have an impact on the outcome of chronic phase CML patients with resistance to several lines of therapy, in situations in which an allogenic stem cell transplantation can not be performed. The aim will be to improve cytogenetic and molecular responses in patients with BCR-ABL dependent (mutations of ABL kinase domain, such as T315I or other) or, in particular, independent mechanisms of resistance. Moreover, associations of ARDAP compounds with more potent TKIs, such as third generation ponatinib, could provide a therapeutic strategy for patients in accelerated/blast phase, with the aim of improving the rates of responses and median overall survival or as a valid bridge to transplant procedures.
[1] Milojkovic, D. et al. Clin. Cancer Res. 2009, 15, 7519-7527. [2]. Jamieson, C. H. M. et al. N. Engl. J. Med. 2004, 351, 657-667. [3] de Bruin, E. C. et al. Cancer Treat. Rev. 2008, 34, 737-749. [4] Teicher, B. A. et al. Clin. Cancer Res. 2008, 14, 1610-1617. [5]. La Regina, G. et al. ACS Med. Chem. Lett. 2017, 8, 521-526.