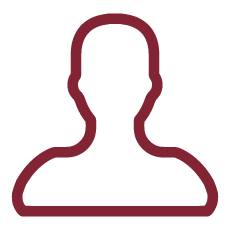
Collective effects due to the electromagnetic interaction of charged beams with a particle accelerator have detrimental effects on the beam quality and have been identified to be potential bottleneck for proposed or planned future accelerators, such as the project High Luminosity LHC and the Future Circular Collider. Moreover, also novel accelerator techniques, such as particle driven plasma accelerators, may suffer of collective effects for high current beams.
The scientific aim of this proposal is to afford the electromagnetic study of innovative devices that will be installed inside these future accelerators, to determine their effect on the beam dynamics, and to find mitigation techniques to reduce the impact of such devices on the beam quality and stability.
The state of the art in the field of particle accelerators is progressing continuously. The accelerators involved in this proposal are very ambitious projects demanding for technology which, in some cases, has still to be developed.
For the HL-LHC project, the overall design and the time schedule is well planned, but many new devices have to be fully analyzed. Our aim is to give a fundamental contribution to determine their electromagnetic impact and influence on the beam dynamics. Our university group is internationally recognized as an expert team of beam dynamics and collective effects, and a fruitful collaboration with CERN is in place since many years, as also the CV of the PI and the other group components shows. The innovation of the research project for HL-LHC is the final design of an important component of the future LHC machine, that is the goniometer for crystal-based collimations. Since several of these devices are planned to be installed, it is very important to provide estimates of the impedance budget, also by considering different types of design, and to elaborate possible mitigation measures in case of problems. The study can be used as reference for other accelerators.
Moreover, by working in collaboration with the Beam Department and the Engineering Department of CERN, we will serve as a link connecting two different aspects of a particle accelerator: the thermo-mechanical design of a device (as the TDIS) and its impact on beam dynamics due to the electromagnetic interaction with the charged beam.
Concerning the FCC project, this new accelerator complex represents a great challenge under several aspects, which requires a strong R&D program on beam dynamics and new technologies. CERN, in collaboration with international institutes, laboratories and universities worldwide, included Sapienza, started the design studies of this new accelerator project in a global context, which necessitates of a vigorous development of new technologies, as, for example, high-field magnets and high-gradient accelerating structures. In this scenario, our group, which is in charge of the collective effects for the FCC-ee collider, will be involved in the choices of new devices having a small impact on the beam dynamics. The reduction of the impedance budget for this huge machine with a circumference of 100 km is of primary importance. The study of the electromagnetic fields of some critical devices of FCC-ee, their effects on the beams dynamics and cures for possible instabilities, represent the state of the art in this field, making also necessary to find innovative solution.
Finally, the charged particle acceleration based on the plasma interaction is a novel technology allowing to generate and support electric fields up to few hundreds of GV/m, thus giving the possibility to accelerate a particle beam to energies up to few GeV in a distance of few cm's instead of km's. In the field of particle driven plasma accelerators, longitudinal wakefields, responsible of the acceleration, are extensively studied in literature. Much less studied are the transverse effects, which may occur when a plasma is confined with external structures, such as capillaries, and the beam is injected off axis.
The problem of transverse wakefields in dielectric capillaries is relevant in most of the modern plasma accelerators, including the laser driven schemes where capillaries are used to enhance the interaction between plasma and external driving laser. Moreover, a particle beam travelling in a dielectric capillary generates Cherenkov radiation in terms of waveguide modes that can propagate inside the pipe and resonantly interact with the beam. In this case, the beam generates relatively narrow-band radiation coming out from the exit of the pipe in the direction of beam propagation. It can be shown that such radiation can be in the THz range, thus very interesting in many applied physics issues. The radiation energy scales with the square of the beam energy, it is proportional to the length of the pipe and, in principle, it can be larger than in the case of conventional schemes for THz radiation generation (e.g. the ones based on beam interaction with a screen). Unfortunately, the duration of the radiation pulse is long, so the energy density is low.
Such limitation can be overcome injecting in the capillary an off-axis beam. The beam possesses a pancake-like electromagnetic field that extends in radial direction several orders of magnitude of the size of the channel. With the help of a standard setup using a metal foil in the path of the beam, this field can be converted into an intense, short, linearly polarized pulse of THz radiation. Focusing this pulse on a sample would allow achieving record electric fields for many applications. However, due to the off-axis injection, strong transverse wakefields may negatively affect the operation of the system.